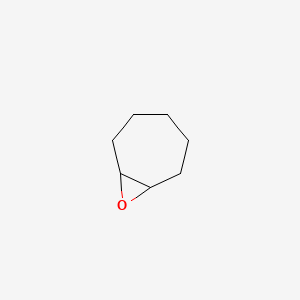
1,2-Epoxycycloheptane
Overview
Description
1,2-Epoxycycloheptane, also known as cycloheptane, 1,2-epoxy-, is a bicyclic organic compound with the molecular formula C₇H₁₂O. It is characterized by a seven-membered ring containing an oxygen atom, forming an epoxide group. This compound is known for its unique structure and reactivity, making it a subject of interest in various fields of chemistry .
Mechanism of Action
Target of Action
This compound, also known as 8-Oxabicyclo[5.1.0]octane, is a type of epoxide, which are highly reactive compounds often involved in various chemical reactions .
Mode of Action
Epoxides like 1,2-Epoxycycloheptane are known for their reactivity, which allows them to interact with a variety of biological targets. They can undergo reactions such as ring-opening, leading to various chemical transformations . .
Biochemical Pathways
Epoxides are generally involved in a variety of biochemical processes due to their reactivity
Pharmacokinetics
It is known that the compound has a molecular weight of 11217 , which could influence its bioavailability and pharmacokinetic properties.
Action Environment
Environmental factors can significantly influence the action, efficacy, and stability of chemical compounds. Factors such as temperature, pH, and the presence of other chemicals can affect the reactivity and stability of epoxides like this compound . .
Preparation Methods
Synthetic Routes and Reaction Conditions
1,2-Epoxycycloheptane can be synthesized through several methods. One efficient method involves the gold(I)-catalyzed tandem 1,3-acyloxy migration/Ferrier rearrangement of glycal-derived 1,6-enyne bearing propargylic carboxylates . This method allows for the preparation of enantiomerically pure 8-oxabicyclo[3.2.1]octanes, which can then undergo further reactions to form the desired compound.
Industrial Production Methods
Industrial production methods for this compound are not well-documented in the literature. the synthesis methods mentioned above can be scaled up for industrial applications, provided that the reaction conditions are optimized for larger-scale production.
Chemical Reactions Analysis
Types of Reactions
1,2-Epoxycycloheptane undergoes various chemical reactions, including oxidation, reduction, and substitution reactions. These reactions are facilitated by the presence of the epoxide group, which is highly reactive.
Common Reagents and Conditions
Oxidation: The compound can be oxidized using reagents such as tempo oxoammonium tetrafluoroborate (T+BF₄⁻) and ZnBr₂.
Reduction: Reduction reactions can be carried out using common reducing agents like lithium aluminum hydride (LiAlH₄).
Substitution: Nucleophilic substitution reactions can occur at the epoxide ring, often using nucleophiles such as amines or thiols.
Major Products
The major products formed from these reactions depend on the specific reagents and conditions used. For example, oxidation can lead to the formation of hydroxylated derivatives, while reduction can yield alcohols.
Scientific Research Applications
1,2-Epoxycycloheptane has several applications in scientific research:
Chemistry: It is used as a building block in the synthesis of more complex organic molecules.
Medicine: Some derivatives of this compound are being investigated for their potential therapeutic applications.
Industry: The compound is used in the development of new materials and as a precursor in various chemical processes.
Comparison with Similar Compounds
1,2-Epoxycycloheptane can be compared with other similar compounds, such as:
Cycloheptene oxide: Another epoxide with a seven-membered ring, but with different reactivity and applications.
Cyclohexene oxide: A six-membered ring epoxide with similar reactivity but different physical and chemical properties.
Cyclooctene oxide: An eight-membered ring epoxide with distinct reactivity and applications.
Properties
IUPAC Name |
8-oxabicyclo[5.1.0]octane | |
---|---|---|
Source | PubChem | |
URL | https://pubchem.ncbi.nlm.nih.gov | |
Description | Data deposited in or computed by PubChem | |
InChI |
InChI=1S/C7H12O/c1-2-4-6-7(8-6)5-3-1/h6-7H,1-5H2 | |
Source | PubChem | |
URL | https://pubchem.ncbi.nlm.nih.gov | |
Description | Data deposited in or computed by PubChem | |
InChI Key |
MLOZFLXCWGERSM-UHFFFAOYSA-N | |
Source | PubChem | |
URL | https://pubchem.ncbi.nlm.nih.gov | |
Description | Data deposited in or computed by PubChem | |
Canonical SMILES |
C1CCC2C(O2)CC1 | |
Source | PubChem | |
URL | https://pubchem.ncbi.nlm.nih.gov | |
Description | Data deposited in or computed by PubChem | |
Molecular Formula |
C7H12O | |
Source | PubChem | |
URL | https://pubchem.ncbi.nlm.nih.gov | |
Description | Data deposited in or computed by PubChem | |
DSSTOX Substance ID |
DTXSID60951266 | |
Record name | 8-Oxabicyclo[5.1.0]octane | |
Source | EPA DSSTox | |
URL | https://comptox.epa.gov/dashboard/DTXSID60951266 | |
Description | DSSTox provides a high quality public chemistry resource for supporting improved predictive toxicology. | |
Molecular Weight |
112.17 g/mol | |
Source | PubChem | |
URL | https://pubchem.ncbi.nlm.nih.gov | |
Description | Data deposited in or computed by PubChem | |
CAS No. |
286-45-3 | |
Record name | 8-Oxabicyclo[5.1.0]octane | |
Source | CAS Common Chemistry | |
URL | https://commonchemistry.cas.org/detail?cas_rn=286-45-3 | |
Description | CAS Common Chemistry is an open community resource for accessing chemical information. Nearly 500,000 chemical substances from CAS REGISTRY cover areas of community interest, including common and frequently regulated chemicals, and those relevant to high school and undergraduate chemistry classes. This chemical information, curated by our expert scientists, is provided in alignment with our mission as a division of the American Chemical Society. | |
Explanation | The data from CAS Common Chemistry is provided under a CC-BY-NC 4.0 license, unless otherwise stated. | |
Record name | 8-Oxabicyclo(5.1.0)octane | |
Source | ChemIDplus | |
URL | https://pubchem.ncbi.nlm.nih.gov/substance/?source=chemidplus&sourceid=0000286453 | |
Description | ChemIDplus is a free, web search system that provides access to the structure and nomenclature authority files used for the identification of chemical substances cited in National Library of Medicine (NLM) databases, including the TOXNET system. | |
Record name | 8-Oxabicyclo[5.1.0]octane | |
Source | EPA DSSTox | |
URL | https://comptox.epa.gov/dashboard/DTXSID60951266 | |
Description | DSSTox provides a high quality public chemistry resource for supporting improved predictive toxicology. | |
Record name | 8-oxabicyclo[5.1.0]octane | |
Source | European Chemicals Agency (ECHA) | |
URL | https://echa.europa.eu/substance-information/-/substanceinfo/100.005.464 | |
Description | The European Chemicals Agency (ECHA) is an agency of the European Union which is the driving force among regulatory authorities in implementing the EU's groundbreaking chemicals legislation for the benefit of human health and the environment as well as for innovation and competitiveness. | |
Explanation | Use of the information, documents and data from the ECHA website is subject to the terms and conditions of this Legal Notice, and subject to other binding limitations provided for under applicable law, the information, documents and data made available on the ECHA website may be reproduced, distributed and/or used, totally or in part, for non-commercial purposes provided that ECHA is acknowledged as the source: "Source: European Chemicals Agency, http://echa.europa.eu/". Such acknowledgement must be included in each copy of the material. ECHA permits and encourages organisations and individuals to create links to the ECHA website under the following cumulative conditions: Links can only be made to webpages that provide a link to the Legal Notice page. | |
Synthesis routes and methods
Procedure details
Retrosynthesis Analysis
AI-Powered Synthesis Planning: Our tool employs the Template_relevance Pistachio, Template_relevance Bkms_metabolic, Template_relevance Pistachio_ringbreaker, Template_relevance Reaxys, Template_relevance Reaxys_biocatalysis model, leveraging a vast database of chemical reactions to predict feasible synthetic routes.
One-Step Synthesis Focus: Specifically designed for one-step synthesis, it provides concise and direct routes for your target compounds, streamlining the synthesis process.
Accurate Predictions: Utilizing the extensive PISTACHIO, BKMS_METABOLIC, PISTACHIO_RINGBREAKER, REAXYS, REAXYS_BIOCATALYSIS database, our tool offers high-accuracy predictions, reflecting the latest in chemical research and data.
Strategy Settings
Precursor scoring | Relevance Heuristic |
---|---|
Min. plausibility | 0.01 |
Model | Template_relevance |
Template Set | Pistachio/Bkms_metabolic/Pistachio_ringbreaker/Reaxys/Reaxys_biocatalysis |
Top-N result to add to graph | 6 |
Feasible Synthetic Routes
Q1: What are the common synthetic routes for producing 8-Oxabicyclo[5.1.0]octane?
A1: 8-Oxabicyclo[5.1.0]octane can be synthesized through the epoxidation of cycloheptene. One study utilized meta-chloroperbenzoic acid (m-CPBA) in dichloromethane at room temperature to achieve this transformation []. This method provides a straightforward approach to obtaining the desired bicyclic epoxide.
Q2: Are there any computational studies investigating the thermodynamic properties of 8-Oxabicyclo[5.1.0]octane?
A2: Yes, density functional theory (DFT) calculations have been employed to determine the thermodynamic properties of 8-Oxabicyclo[5.1.0]octane. Specifically, the B3LYP/6-31G(d,p) level of theory was used to calculate its enthalpy of formation (ΔfH°(298)), standard entropy (S°(298)), and heat capacity [Cp°(T)] over a temperature range of 10K to 5000K [, ]. These studies provide valuable insights into the stability and reactivity of this compound.
Q3: How does the structure of 8-Oxabicyclo[5.1.0]octane relate to its potential applications?
A3: The strained bicyclic structure of 8-Oxabicyclo[5.1.0]octane, particularly the epoxide ring, makes it a reactive species susceptible to ring-opening reactions. This reactivity makes it a valuable building block in organic synthesis. For instance, it can be employed as a precursor for synthesizing more complex molecules, such as pharmaceuticals and polymers [].
Disclaimer and Information on In-Vitro Research Products
Please be aware that all articles and product information presented on BenchChem are intended solely for informational purposes. The products available for purchase on BenchChem are specifically designed for in-vitro studies, which are conducted outside of living organisms. In-vitro studies, derived from the Latin term "in glass," involve experiments performed in controlled laboratory settings using cells or tissues. It is important to note that these products are not categorized as medicines or drugs, and they have not received approval from the FDA for the prevention, treatment, or cure of any medical condition, ailment, or disease. We must emphasize that any form of bodily introduction of these products into humans or animals is strictly prohibited by law. It is essential to adhere to these guidelines to ensure compliance with legal and ethical standards in research and experimentation.