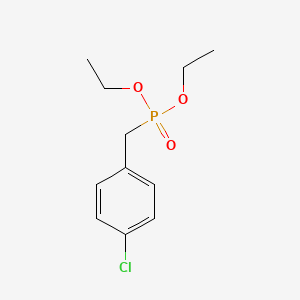
Diethyl 4-chlorobenzylphosphonate
Overview
Description
Diethyl 4-chlorobenzylphosphonate (CAS 39225-17-7) is an organophosphonate compound with the molecular formula C₁₁H₁₆ClO₃P and a molar mass of 262.67 g/mol . Its structure features a benzyl group substituted with a chlorine atom at the para position, linked to a diethyl phosphonate group. Key physical properties include a density of 1.19 g/mL at 25°C and applications in organic synthesis as an intermediate for coupling reactions .
Synthesis typically follows the Abramov or Pudovik reactions, utilizing hydrophosphoryl reagents derived from hypophosphorous acids and alcohols, a cost-effective industrial process .
Preparation Methods
Direct Esterification of Phosphonic Acids
One of the classical methods involves the esterification of the corresponding phosphonic acid with triethyl orthoacetate under heating conditions. For example, benzylphosphonic acid derivatives can be reacted with triethyl orthoacetate at elevated temperatures (~90 °C) overnight to form diethyl benzylphosphonates. This method has been reported for general benzylphosphonate esters and can be adapted for the 4-chloro-substituted analogue.
Reaction Conditions:
- Starting material: 4-chlorobenzylphosphonic acid (1 mmol)
- Reagent: Triethyl orthoacetate (excess, e.g., 30 mmol)
- Temperature: 90 °C
- Time: Overnight
- Monitoring: Reaction progress monitored by appropriate analytical methods
This method yields the diethyl ester by selective esterification of the phosphonic acid group.
Coupling of Arylboronic Acids with Phosphonate Esters
An advanced and more efficient approach to synthesize diethyl 4-chlorobenzylphosphonate involves palladium-catalyzed coupling reactions of arylboronic acids bearing phosphonate ester moieties with vinyl esters, followed by further transformations.
- The use of 4-(diethoxyphosphoryl)methylphenylboronic acid as a coupling partner improves reaction efficiency.
- This approach overcomes the low yields of earlier methods that relied on coupling p-hydroxymethylphenylboronic acid with olefins followed by Arbuzov reaction.
- The yield improvement is significant, with reported yields increasing from about 1% to approximately 38% for related compounds.
Typical Reaction Scheme:
- Pd-catalyzed coupling of diethyl (4-(4,4,5,5-tetramethyl-1,3,2-dioxaborolan-2-yl)benzyl)phosphonate with vinyl acetate
- Formation of intermediates such as 4-((diethoxyphosphoryl)methyl)styryl acetate
- Subsequent transformations yield diethyl benzylphosphonate derivatives including the 4-chloro-substituted compound
Michaelis–Arbuzov Reaction and Variants
The Michaelis–Arbuzov reaction is a classical method for preparing alkyl phosphonates by reacting alkyl halides with trialkyl phosphites. However, for α-halo-benzylphosphonates, this reaction is often inefficient.
- α-Chloro- or α-bromo-benzylphosphonates are generally poor substrates for the Michaelis–Arbuzov reaction.
- A novel approach involves the use of α-methanesulfonyloxy-benzylphosphonates, which undergo efficient Arbuzov fission with triethyl phosphite at elevated temperatures (around 135 °C), yielding arylmethylenebisphosphonates in good yields (76–81%).
Preparation via α-Hydroxybenzylphosphonates and Subsequent Functionalization
This compound can also be prepared starting from diethyl α-hydroxy-4-chlorobenzylphosphonate, which is synthesized by condensation of diethyl phosphite with the corresponding aldehyde under basic conditions.
- The α-hydroxybenzylphosphonates can be acylated or sulfonylated to introduce further functionality.
- Acylation with acyl chlorides (e.g., acetyl chloride) in the presence of triethylamine in toluene at temperatures between 25 °C and 60 °C provides acylated derivatives in high yields (69–97%).
- Sulfonylation with methanesulfonyl chloride or p-toluenesulfonyl chloride in the presence of triethylamine yields mesylated or tosylated derivatives in 54–80% yields, which can be further transformed into the desired phosphonate esters.
Table 1: Representative Yields of Sulfonylated α-Hydroxybenzylphosphonates
Entry | Starting Material Substituent | Sulfonyl Chloride | Reaction Time (h) | Product | Yield (%) |
---|---|---|---|---|---|
1 | 4-H | Methanesulfonyl | 0.5 | Mesylate 4a | 67 |
2 | 3,5-di-tert-butyl | Methanesulfonyl | 0.5 | Mesylate 4b | 60 |
4 | 4-Cl | Methanesulfonyl | 0.5 | Mesylate 4e | 66 |
12 | 4-Cl | p-Toluenesulfonyl | 0.5 | Tosylate 6a | 65 |
Note: The 4-chloro substituent corresponds to the target compound's aromatic ring.
Summary of Key Preparation Methods
Method | Starting Materials | Reaction Conditions | Yield Range (%) | Notes |
---|---|---|---|---|
Direct esterification | 4-Chlorobenzylphosphonic acid + triethyl orthoacetate | 90 °C, overnight | Moderate | Classical method, straightforward |
Pd-catalyzed coupling | Arylboronic acid with phosphonate ester + vinyl acetate | Pd catalyst, mild conditions | Up to 38% | Improved efficiency over older methods |
Michaelis–Arbuzov variant | α-Methanesulfonyloxy-benzylphosphonates + triethyl phosphite | 135 °C, excess phosphite | 76–81% | Efficient Arbuzov fission |
Acylation of α-hydroxybenzylphosphonates | α-Hydroxybenzylphosphonates + acyl chlorides + triethylamine | 25–60 °C, toluene | 69–97% | Provides acylated intermediates |
Sulfonylation of α-hydroxybenzylphosphonates | α-Hydroxybenzylphosphonates + methanesulfonyl or p-toluenesulfonyl chloride + triethylamine | 25 °C, 0.5 h, toluene | 54–80% | Yields mesylated/tosylated derivatives |
Research Findings and Analytical Data
- The esterification and coupling reactions are monitored by nuclear magnetic resonance (NMR) spectroscopy, including ^31P, ^13C, and ^1H NMR, confirming the formation of the phosphonate esters.
- High-resolution mass spectrometry (HRMS) is used to verify molecular weights and purity.
- The sulfonylation reactions proceed with high selectivity and good yields, with the 4-chloro substituent influencing reaction conditions moderately.
- Acylation reactions require slightly elevated temperatures for electron-withdrawing substituents like 4-chloro to achieve complete conversion.
- The improved coupling method using arylboronic acids with phosphonate esters represents a significant advancement in synthetic efficiency for these compounds.
Chemical Reactions Analysis
Diethyl 4-chlorobenzylphosphonate undergoes various chemical reactions, including:
Substitution Reactions: It can participate in nucleophilic substitution reactions, where the chlorine atom is replaced by other nucleophiles.
Oxidation and Reduction: The compound can be oxidized or reduced under specific conditions, leading to different products.
Wittig-Horner Reactions: It is used in Wittig-Horner reactions to form alkenes.
Common reagents used in these reactions include sodium borohydride for reduction and potassium permanganate for oxidation. The major products formed depend on the specific reaction conditions and reagents used.
Scientific Research Applications
Organic Synthesis
Diethyl 4-chlorobenzylphosphonate is primarily utilized as a reagent in various organic synthesis reactions:
- Preparation of Chromenone and Chromene Derivatives : It serves as a precursor for synthesizing chromenone and chromene derivatives, which have applications as anti-picornavirus capsid-binders .
- Wittig-Horner Reactions : This compound is used in Wittig-Horner reactions to create phosphonates that are significant in the synthesis of complex organic molecules .
- Asymmetric Synthesis of Gamma-Nitrophosphonates : It is involved in the Michael addition with nitroalkenes, facilitating the synthesis of gamma-nitrophosphonates .
Medicinal Chemistry
Recent studies have highlighted the potential anticancer properties of this compound derivatives:
- Cytotoxicity Against Cancer Cell Lines : In a study involving acylated dialkyl α-hydroxy-benzylphosphonates, compounds derived from this compound exhibited moderate cytostatic effects against various cancer cell lines, including breast, prostate, and lung carcinomas . The compounds demonstrated dose-dependent cytotoxicity, indicating their potential as anticancer agents.
Cell Line | Cytostatic Effect (%) |
---|---|
MDA-MB-231 (Breast) | 29.7 ± 4.7 |
A431 (Skin) | 30.5 ± 2.6 |
PC-3 (Prostate) | 40.8 ± 4.8 |
HT-29 (Colon) | <30 |
Analytical Applications
This compound is also employed in analytical chemistry:
- HPLC Analysis : It can be separated and analyzed using reverse-phase high-performance liquid chromatography (HPLC). The method utilizes a mobile phase consisting of acetonitrile, water, and phosphoric acid, making it suitable for isolating impurities and pharmacokinetic studies .
Biochemical Applications
This compound finds applications in various biochemical processes:
- Cell Culture and Gene Therapy : It is utilized in bioprocessing and cell culture applications, contributing to advancements in gene therapy techniques .
- Molecular Testing : this compound is involved in molecular testing methodologies that are critical for diagnostics and therapeutic development .
Case Study 1: Anticancer Activity
A comprehensive study assessed the cytotoxic effects of diethyl α-hydroxy-benzylphosphonates against eight tumor cell lines at a concentration of 50 µM. The results indicated that certain derivatives were particularly effective against multidrug-resistant cancer cells, suggesting that modifications to the phosphonate structure could enhance therapeutic efficacy .
Case Study 2: HPLC Method Development
A method was developed for the separation of this compound using HPLC on Newcrom R1 columns. This method demonstrated scalability for preparative separations, making it valuable for both analytical and preparative applications in pharmaceutical research .
Mechanism of Action
The mechanism of action of diethyl 4-chlorobenzylphosphonate involves its ability to inhibit cell proliferation by binding to specific receptors and inducing apoptosis in cells . It also inhibits angiogenesis, leading to decreased microvessel density and endothelial cell proliferation in tumor cells . This makes it a potential candidate for cancer treatment.
Comparison with Similar Compounds
Comparison with Structural Analogs
Diethyl 4-Cyanobenzylphosphonate
- Molecular Formula: C₁₂H₁₆NO₃P
- Molar Mass : 253.238 g/mol
- CAS : 1552-41-6
- Key Features: The para-cyano (-CN) group is a strong electron-withdrawing substituent, enhancing the electrophilicity of the benzyl carbon. This increases reactivity in nucleophilic substitutions compared to the chloro analog. Applications may include coordination chemistry or as a precursor for bioactive molecules.
Diethyl 4-Methoxybenzylphosphonate
- Molecular Formula : C₁₂H₁₉O₄P
- Molar Mass : 258.254 g/mol
- CAS : 1145-93-3
- Key Features: The methoxy (-OCH₃) group is electron-donating, reducing the electrophilicity of the benzyl carbon. This may lower reactivity in coupling reactions but improve solubility in polar solvents. Potential uses include agrochemical intermediates.
Diethyl 4-Bromobenzylphosphonate
- Molecular Formula : C₁₁H₁₆BrO₃P
- CAS : 38186-51-5
- However, its similar electronegativity means reactivity in radical or halogen-exchange reactions may align with the chloro derivative .
Diethyl 4-(Trifluoromethyl)benzylphosphonate
- Molecular Formula : C₁₂H₁₅F₃O₃P
- CAS: Not explicitly listed (see )
- Key Features : The trifluoromethyl (-CF₃) group is highly electron-withdrawing, significantly increasing the phosphonate’s acidity. This enhances its utility in palladium-catalyzed cross-couplings or as a ligand in catalysis .
Diethyl [4-(Diphenylamino)benzyl]phosphonate
- Molecular Formula: C₂₂H₂₅NO₃P
- CAS: Not explicitly listed (see )
Comparative Data Table
Research Findings and Substituent Effects
- Electronic Effects : Electron-withdrawing groups (e.g., -CN, -CF₃) enhance the electrophilicity of the benzyl carbon, favoring nucleophilic attack in reactions like Michael additions or cross-couplings. Conversely, electron-donating groups (e.g., -OCH₃) reduce reactivity but improve solubility .
- Steric Effects : Bulkier substituents (e.g., -Br, -NPh₂) may hinder reaction rates in sterically demanding environments but can stabilize transition states in specific catalytic cycles .
- Biological Activity : The chloro derivative’s antiviral efficacy () suggests halogen size and electronegativity are critical for target binding, though substituent-specific studies are needed.
Biological Activity
Diethyl 4-chlorobenzylphosphonate (DECBP) is an organophosphorus compound that has garnered attention for its potential biological activities, particularly in the fields of antimicrobial and anticancer research. Its structure, characterized by a chlorobenzyl moiety attached to a diethyl phosphonate group, suggests diverse interactions with biological systems. This article reviews the biological activity of DECBP, supported by various studies, including antimicrobial efficacy and cytotoxic effects against cancer cell lines.
This compound is synthesized through electrophilic halogenation of diethyl benzylphosphonate. The compound can be represented as follows:
- Molecular Formula : C11H16ClO3P
- Molecular Weight : 251.67 g/mol
Synthesis
The synthesis of DECBP involves the reaction of diethyl benzylphosphonate with chlorinating agents under controlled conditions to achieve high yields and purity. Analytical techniques such as NMR spectroscopy are employed to confirm the structure and purity of the synthesized compound .
Antimicrobial Activity
Recent studies have demonstrated that diethyl benzylphosphonate derivatives, including DECBP, exhibit significant antimicrobial properties against various bacterial strains. Notably, DECBP was tested against Escherichia coli strains, showing a low minimal inhibitory concentration (MIC), indicating its potential as an antimicrobial agent . The results suggest that the introduction of different substituents on the phenyl ring can modulate the antimicrobial efficacy.
Table 1: Antimicrobial Activity of this compound
Compound | Bacterial Strain | MIC (µg/mL) | MBC (µg/mL) |
---|---|---|---|
This compound | E. coli K12 | 32 | 64 |
Diethyl benzylphosphonate | E. coli R2-R4 | 64 | 128 |
Cytotoxicity and Anticancer Activity
In vitro studies have assessed the cytotoxic effects of DECBP on various cancer cell lines, including MDA-MB-231 (breast cancer) and A431 (epidermoid carcinoma). The compound exhibited dose-dependent cytotoxicity, with significant antiproliferative effects noted at concentrations as low as 50 µM .
Table 2: Cytotoxic Effects of this compound on Cancer Cell Lines
Cell Line | Concentration (µM) | % Viability |
---|---|---|
MDA-MB-231 | 50 | 36.1 |
A431 | 50 | 40.8 |
PC-3 | 50 | 29.7 |
The mechanism underlying the biological activity of DECBP appears to involve oxidative stress induction leading to DNA damage in bacterial cells. Studies indicated that treatment with DECBP resulted in significant alterations in bacterial DNA topology, suggesting that oxidative damage plays a crucial role in its antimicrobial action . Furthermore, the cytotoxicity observed in cancer cells may be attributed to apoptosis induction and interference with cellular signaling pathways.
Case Studies
- Antimicrobial Study : A study conducted on various E. coli strains revealed that DECBP caused significant growth inhibition, with a notable reduction in viability correlating with increased concentrations of the compound.
- Cytotoxicity Assessment : In a comparative analysis involving several phosphonates, DECBP demonstrated superior cytotoxicity against multidrug-resistant cancer cell lines, highlighting its potential as a therapeutic agent for resistant cancers .
Q & A
Basic Research Questions
Q. What are the optimal synthetic routes for preparing diethyl 4-chlorobenzylphosphonate, and how can reaction conditions be systematically optimized?
this compound is typically synthesized via nucleophilic substitution or Arbuzov-type reactions. A common method involves reacting 4-chlorobenzyl chloride with triethyl phosphite under controlled heating (80–120°C). Optimization requires monitoring reaction time, temperature, and stoichiometry using thin-layer chromatography (TLC) or GC-MS. For scalability, highlights the use of Grignard reagents (e.g., isopropylmagnesium chloride) to reduce byproducts. Post-synthesis purification via vacuum distillation or column chromatography (silica gel, ethyl acetate/hexane eluent) is critical to achieve >95% purity .
Q. How do solubility properties of this compound influence its application in organic synthesis?
The compound is soluble in polar aprotic solvents (e.g., dichloromethane, acetone) but insoluble in water, as noted in and . This solubility profile makes it suitable for reactions in non-aqueous media, such as Horner-Wadsworth-Emmons (HWE) olefinations. Researchers should pre-dry solvents to avoid hydrolysis of the phosphonate ester. For aqueous-phase applications, phase-transfer catalysts or micellar systems may be required .
Q. What safety protocols are essential for handling this compound in laboratory settings?
While the compound is not classified as highly hazardous, and emphasize standard precautions: use PPE (gloves, goggles), work in a fume hood, and avoid inhalation/contact. Spills should be neutralized with inert adsorbents (e.g., vermiculite) and disposed of as halogenated waste. Stability tests indicate it is incompatible with strong oxidizers; store in airtight containers at 2–8°C to prevent degradation .
Advanced Research Questions
Q. How does the para-chloro substituent affect the reactivity of this compound in cross-coupling reactions compared to other substituents (e.g., bromo, cyano)?
The electron-withdrawing chloro group enhances the electrophilicity of the benzyl carbon, facilitating nucleophilic attacks in HWE reactions. and demonstrate that chloro-substituted phosphonates exhibit slower reaction kinetics than bromo analogs but higher selectivity in forming trans-alkenes. Computational studies (DFT) can model substituent effects on transition states, while comparative kinetic experiments (e.g., with diethyl 4-bromobenzylphosphonate) quantify reactivity differences .
Q. What analytical techniques are most effective for resolving contradictions in spectroscopic data (e.g., NMR splitting patterns) for this compound derivatives?
Contradictions in P NMR or H NMR splitting may arise from rotameric equilibria or impurities. High-field NMR (≥400 MHz) with decoupling and variable-temperature experiments can resolve overlapping signals. For example, cooling to –40°C slows rotation around the P–C bond, simplifying splitting patterns. Mass spectrometry (HRMS) and X-ray crystallography (if crystalline) validate structural assignments .
Q. How can this compound be leveraged in designing metal-organic frameworks (MOFs) or surface-modified materials?
The phosphonate group acts as a chelating agent for metal ions (e.g., Zr, Ti) in MOF synthesis. and suggest that the chloro substituent introduces hydrophobicity, useful for gas adsorption or catalytic sites. Surface modification protocols involve sonicating the compound with metal oxides (e.g., TiO) in ethanol, followed by thermal annealing. XPS and BET surface area analysis characterize functionalization efficiency .
Q. What mechanisms underlie the reported biological activity of this compound derivatives in antiviral studies?
indicates benzylphosphonates inhibit viral proteases or polymerases via phosphonate-metal coordination (e.g., binding Mg in active sites). To validate, researchers can perform enzyme inhibition assays (e.g., HIV-1 protease) with IC measurements and molecular docking simulations. Comparative studies with non-chlorinated analogs (e.g., diethyl benzylphosphonate) isolate the role of the chloro group in bioactivity .
Q. Methodological Tables
Q. Table 1: Comparative Reactivity of Substituted Benzylphosphonates
Substituent | Reaction Rate (HWE Olefination) | Selectivity (trans:cis) | Reference |
---|---|---|---|
4-Cl | Moderate (k = 0.45 Ms) | 85:15 | |
4-Br | Fast (k = 0.78 Ms) | 70:30 | |
4-CN | Slow (k = 0.22 Ms) | 95:5 |
Q. Table 2: Safety and Storage Guidelines
Parameter | Recommendation | Source |
---|---|---|
Storage Temperature | 2–8°C (protect from light) | |
Incompatible Substances | Strong oxidizers (e.g., HNO, KMnO) | |
PPE | Nitrile gloves, chemical goggles |
Properties
IUPAC Name |
1-chloro-4-(diethoxyphosphorylmethyl)benzene | |
---|---|---|
Source | PubChem | |
URL | https://pubchem.ncbi.nlm.nih.gov | |
Description | Data deposited in or computed by PubChem | |
InChI |
InChI=1S/C11H16ClO3P/c1-3-14-16(13,15-4-2)9-10-5-7-11(12)8-6-10/h5-8H,3-4,9H2,1-2H3 | |
Source | PubChem | |
URL | https://pubchem.ncbi.nlm.nih.gov | |
Description | Data deposited in or computed by PubChem | |
InChI Key |
BVQPVBZRJSFOEZ-UHFFFAOYSA-N | |
Source | PubChem | |
URL | https://pubchem.ncbi.nlm.nih.gov | |
Description | Data deposited in or computed by PubChem | |
Canonical SMILES |
CCOP(=O)(CC1=CC=C(C=C1)Cl)OCC | |
Source | PubChem | |
URL | https://pubchem.ncbi.nlm.nih.gov | |
Description | Data deposited in or computed by PubChem | |
Molecular Formula |
C11H16ClO3P | |
Source | PubChem | |
URL | https://pubchem.ncbi.nlm.nih.gov | |
Description | Data deposited in or computed by PubChem | |
DSSTOX Substance ID |
DTXSID70192486 | |
Record name | Diethyl 4-chlorobenzylphosphonate | |
Source | EPA DSSTox | |
URL | https://comptox.epa.gov/dashboard/DTXSID70192486 | |
Description | DSSTox provides a high quality public chemistry resource for supporting improved predictive toxicology. | |
Molecular Weight |
262.67 g/mol | |
Source | PubChem | |
URL | https://pubchem.ncbi.nlm.nih.gov | |
Description | Data deposited in or computed by PubChem | |
CAS No. |
39225-17-7 | |
Record name | Diethyl P-[(4-chlorophenyl)methyl]phosphonate | |
Source | CAS Common Chemistry | |
URL | https://commonchemistry.cas.org/detail?cas_rn=39225-17-7 | |
Description | CAS Common Chemistry is an open community resource for accessing chemical information. Nearly 500,000 chemical substances from CAS REGISTRY cover areas of community interest, including common and frequently regulated chemicals, and those relevant to high school and undergraduate chemistry classes. This chemical information, curated by our expert scientists, is provided in alignment with our mission as a division of the American Chemical Society. | |
Explanation | The data from CAS Common Chemistry is provided under a CC-BY-NC 4.0 license, unless otherwise stated. | |
Record name | Diethyl 4-chlorobenzylphosphonate | |
Source | ChemIDplus | |
URL | https://pubchem.ncbi.nlm.nih.gov/substance/?source=chemidplus&sourceid=0039225177 | |
Description | ChemIDplus is a free, web search system that provides access to the structure and nomenclature authority files used for the identification of chemical substances cited in National Library of Medicine (NLM) databases, including the TOXNET system. | |
Record name | 39225-17-7 | |
Source | DTP/NCI | |
URL | https://dtp.cancer.gov/dtpstandard/servlet/dwindex?searchtype=NSC&outputformat=html&searchlist=202843 | |
Description | The NCI Development Therapeutics Program (DTP) provides services and resources to the academic and private-sector research communities worldwide to facilitate the discovery and development of new cancer therapeutic agents. | |
Explanation | Unless otherwise indicated, all text within NCI products is free of copyright and may be reused without our permission. Credit the National Cancer Institute as the source. | |
Record name | Diethyl 4-chlorobenzylphosphonate | |
Source | EPA DSSTox | |
URL | https://comptox.epa.gov/dashboard/DTXSID70192486 | |
Description | DSSTox provides a high quality public chemistry resource for supporting improved predictive toxicology. | |
Record name | Diethyl 4-chlorobenzylphosphonate | |
Source | European Chemicals Agency (ECHA) | |
URL | https://echa.europa.eu/substance-information/-/substanceinfo/100.049.405 | |
Description | The European Chemicals Agency (ECHA) is an agency of the European Union which is the driving force among regulatory authorities in implementing the EU's groundbreaking chemicals legislation for the benefit of human health and the environment as well as for innovation and competitiveness. | |
Explanation | Use of the information, documents and data from the ECHA website is subject to the terms and conditions of this Legal Notice, and subject to other binding limitations provided for under applicable law, the information, documents and data made available on the ECHA website may be reproduced, distributed and/or used, totally or in part, for non-commercial purposes provided that ECHA is acknowledged as the source: "Source: European Chemicals Agency, http://echa.europa.eu/". Such acknowledgement must be included in each copy of the material. ECHA permits and encourages organisations and individuals to create links to the ECHA website under the following cumulative conditions: Links can only be made to webpages that provide a link to the Legal Notice page. | |
Record name | DIETHYL 4-CHLOROBENZYLPHOSPHONATE | |
Source | FDA Global Substance Registration System (GSRS) | |
URL | https://gsrs.ncats.nih.gov/ginas/app/beta/substances/0HV6QW8F6X | |
Description | The FDA Global Substance Registration System (GSRS) enables the efficient and accurate exchange of information on what substances are in regulated products. Instead of relying on names, which vary across regulatory domains, countries, and regions, the GSRS knowledge base makes it possible for substances to be defined by standardized, scientific descriptions. | |
Explanation | Unless otherwise noted, the contents of the FDA website (www.fda.gov), both text and graphics, are not copyrighted. They are in the public domain and may be republished, reprinted and otherwise used freely by anyone without the need to obtain permission from FDA. Credit to the U.S. Food and Drug Administration as the source is appreciated but not required. | |
Retrosynthesis Analysis
AI-Powered Synthesis Planning: Our tool employs the Template_relevance Pistachio, Template_relevance Bkms_metabolic, Template_relevance Pistachio_ringbreaker, Template_relevance Reaxys, Template_relevance Reaxys_biocatalysis model, leveraging a vast database of chemical reactions to predict feasible synthetic routes.
One-Step Synthesis Focus: Specifically designed for one-step synthesis, it provides concise and direct routes for your target compounds, streamlining the synthesis process.
Accurate Predictions: Utilizing the extensive PISTACHIO, BKMS_METABOLIC, PISTACHIO_RINGBREAKER, REAXYS, REAXYS_BIOCATALYSIS database, our tool offers high-accuracy predictions, reflecting the latest in chemical research and data.
Strategy Settings
Precursor scoring | Relevance Heuristic |
---|---|
Min. plausibility | 0.01 |
Model | Template_relevance |
Template Set | Pistachio/Bkms_metabolic/Pistachio_ringbreaker/Reaxys/Reaxys_biocatalysis |
Top-N result to add to graph | 6 |
Feasible Synthetic Routes
Disclaimer and Information on In-Vitro Research Products
Please be aware that all articles and product information presented on BenchChem are intended solely for informational purposes. The products available for purchase on BenchChem are specifically designed for in-vitro studies, which are conducted outside of living organisms. In-vitro studies, derived from the Latin term "in glass," involve experiments performed in controlled laboratory settings using cells or tissues. It is important to note that these products are not categorized as medicines or drugs, and they have not received approval from the FDA for the prevention, treatment, or cure of any medical condition, ailment, or disease. We must emphasize that any form of bodily introduction of these products into humans or animals is strictly prohibited by law. It is essential to adhere to these guidelines to ensure compliance with legal and ethical standards in research and experimentation.