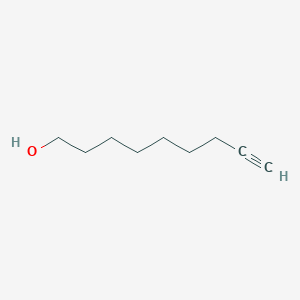
8-Nonyn-1-ol
Overview
Description
Molecular Structure Analysis
The molecular structure of 8-Nonyn-1-ol can be represented by the InChI string:InChI=1S/C9H16O/c1-2-3-4-5-6-7-8-9-10/h1,10H,3-9H2
. The Canonical SMILES representation is C#CCCCCCCCO
. Physical And Chemical Properties Analysis
This compound has a density of 0.9±0.1 g/cm³ . Its boiling point is 212.2±23.0 °C at 760 mmHg . The compound has a vapour pressure of 0.0±0.9 mmHg at 25°C . The enthalpy of vaporization is 52.2±6.0 kJ/mol . The flash point of this compound is 144.6±14.9 °C . The index of refraction is 1.457 . The molar refractivity is 43.1±0.3 cm³ .Scientific Research Applications
Attraction in Moths
8-Nonyn-1-ol is significant in entomology, particularly in the study of moth behavior. For instance, it's closely related to trans-8, trans-10-Dodecadien-1-ol, a sex attractant of the codling moth, Laspeyresia pomonella. Research utilizing electroantennogram techniques has demonstrated the compound's efficacy in attracting male codling moths in the field, indicating its potential application in pest control strategies (Roelofs et al., 1971).
Molecular Dynamics and Charge Transfer
In the field of molecular dynamics, studies have explored the impact of solvent polarity on the lifetime of the low-lying S(1) state of carbonyl-containing polyenes and carotenoids. Research involving this compound derivatives, such as 8,8'-diapocarotene-8'-ol-8-al, has shown how intramolecular charge transfer states are influenced by the number of double bonds in the conjugated π-electron system. These findings have implications in understanding the molecular nature of such states and their role in spectral and dynamic properties of polyenes and carotenoids (Enriquez et al., 2010).
Electroluminescence and OLEDs
Research in the field of organic light-emitting diodes (OLEDs) has investigated the role of compounds structurally similar to this compound. For example, studies on 1,8-naphthalimide derivatives in OLEDs reveal insights into the interplay between dopant, exciplex, and host emission. These findings contribute to the development of more efficient OLEDs, with potential applications in display and lighting technologies (Kolosov et al., 2002).
Synthesis and Structural Investigation
The synthesis and structural investigation of compounds like this compound have implications in various scientific fields. Studies have examined methods for synthesizing related compounds, such as trans-8-oxabicyclo[4.3.0]nonan-3-ols, and investigated their structures using techniques like nuclear magnetic resonance spectroscopy. This research aids in the understanding of molecular structures and has potential applications in chemical synthesis and drug development (Leong et al., 1973).
Corrosion Inhibition and Surface Coatings
This compound derivatives have also been studied in the context of corrosion inhibition and the development of surface coatings. For instance, research on novel organic–inorganic hybrid materials, involving molecules like 1-azanaphthalene-8-ol, has led to the creation of coatings with unique structures and enhanced corrosion stability. These findings have practical applications in materials science and engineering (Kaseem et al., 2021).
Safety and Hazards
When handling 8-Nonyn-1-ol, it is advised to avoid breathing mist, gas or vapours . Contact with skin and eyes should be avoided . Use personal protective equipment and wear chemical impermeable gloves . Ensure adequate ventilation and remove all sources of ignition . In case of accidental release, evacuate personnel to safe areas and keep people away from and upwind of spill/leak .
properties
IUPAC Name |
non-8-yn-1-ol | |
---|---|---|
Source | PubChem | |
URL | https://pubchem.ncbi.nlm.nih.gov | |
Description | Data deposited in or computed by PubChem | |
InChI |
InChI=1S/C9H16O/c1-2-3-4-5-6-7-8-9-10/h1,10H,3-9H2 | |
Source | PubChem | |
URL | https://pubchem.ncbi.nlm.nih.gov | |
Description | Data deposited in or computed by PubChem | |
InChI Key |
XTCPLAOSDRPLKZ-UHFFFAOYSA-N | |
Source | PubChem | |
URL | https://pubchem.ncbi.nlm.nih.gov | |
Description | Data deposited in or computed by PubChem | |
Canonical SMILES |
C#CCCCCCCCO | |
Source | PubChem | |
URL | https://pubchem.ncbi.nlm.nih.gov | |
Description | Data deposited in or computed by PubChem | |
Molecular Formula |
C9H16O | |
Source | PubChem | |
URL | https://pubchem.ncbi.nlm.nih.gov | |
Description | Data deposited in or computed by PubChem | |
DSSTOX Substance ID |
DTXSID60396693 | |
Record name | 8-nonyn-1-ol | |
Source | EPA DSSTox | |
URL | https://comptox.epa.gov/dashboard/DTXSID60396693 | |
Description | DSSTox provides a high quality public chemistry resource for supporting improved predictive toxicology. | |
Molecular Weight |
140.22 g/mol | |
Source | PubChem | |
URL | https://pubchem.ncbi.nlm.nih.gov | |
Description | Data deposited in or computed by PubChem | |
CAS RN |
10160-28-8 | |
Record name | 8-nonyn-1-ol | |
Source | EPA DSSTox | |
URL | https://comptox.epa.gov/dashboard/DTXSID60396693 | |
Description | DSSTox provides a high quality public chemistry resource for supporting improved predictive toxicology. | |
Synthesis routes and methods I
Procedure details
Synthesis routes and methods II
Procedure details
Synthesis routes and methods III
Procedure details
Synthesis routes and methods IV
Procedure details
Retrosynthesis Analysis
AI-Powered Synthesis Planning: Our tool employs the Template_relevance Pistachio, Template_relevance Bkms_metabolic, Template_relevance Pistachio_ringbreaker, Template_relevance Reaxys, Template_relevance Reaxys_biocatalysis model, leveraging a vast database of chemical reactions to predict feasible synthetic routes.
One-Step Synthesis Focus: Specifically designed for one-step synthesis, it provides concise and direct routes for your target compounds, streamlining the synthesis process.
Accurate Predictions: Utilizing the extensive PISTACHIO, BKMS_METABOLIC, PISTACHIO_RINGBREAKER, REAXYS, REAXYS_BIOCATALYSIS database, our tool offers high-accuracy predictions, reflecting the latest in chemical research and data.
Strategy Settings
Precursor scoring | Relevance Heuristic |
---|---|
Min. plausibility | 0.01 |
Model | Template_relevance |
Template Set | Pistachio/Bkms_metabolic/Pistachio_ringbreaker/Reaxys/Reaxys_biocatalysis |
Top-N result to add to graph | 6 |
Feasible Synthetic Routes
Q & A
Q1: What is the significance of 8-Nonyn-1-ol in organic synthesis, and how is it typically synthesized?
A1: this compound serves as a key intermediate in synthesizing various organic compounds, particularly C-16 alkynic fatty acids, which exhibit potent anticancer properties []. A common synthesis route involves the isomerization of internal alkynols, like 2-nonyn-1-ol, using potassium 3-aminopropylamide (KAPA) reagent []. This method enables rapid and efficient conversion to this compound within minutes, achieving yields exceeding 90% [].
Q2: Can you elaborate on the use of this compound in pheromone synthesis, specifically for the oriental fruit moth?
A2: this compound plays a crucial role as a precursor in the synthesis of (Z)-8-Dodecen-1-yl acetate, the primary sex pheromone component of the oriental fruit moth (Grapholitha molesta Busck) []. Researchers utilize a multi-step synthesis pathway starting with this compound to produce this pheromone, which is instrumental in pest management strategies for this agricultural pest [].
Disclaimer and Information on In-Vitro Research Products
Please be aware that all articles and product information presented on BenchChem are intended solely for informational purposes. The products available for purchase on BenchChem are specifically designed for in-vitro studies, which are conducted outside of living organisms. In-vitro studies, derived from the Latin term "in glass," involve experiments performed in controlled laboratory settings using cells or tissues. It is important to note that these products are not categorized as medicines or drugs, and they have not received approval from the FDA for the prevention, treatment, or cure of any medical condition, ailment, or disease. We must emphasize that any form of bodily introduction of these products into humans or animals is strictly prohibited by law. It is essential to adhere to these guidelines to ensure compliance with legal and ethical standards in research and experimentation.