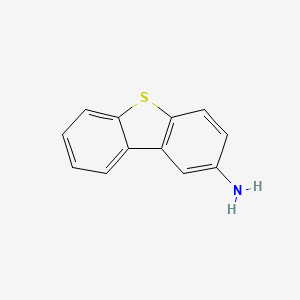
2-Dibenzothiophenamine
Overview
Description
2-Dibenzothiophenamine is a heterocyclic compound with the molecular formula C₁₂H₉NS. It is characterized by a dibenzothiophene core with an amino group attached to the second carbon atom. This compound is of significant interest in various fields of research, including medicinal chemistry, material science, and organic synthesis .
Preparation Methods
Synthetic Routes and Reaction Conditions: 2-Dibenzothiophenamine can be synthesized through several methods. One common approach involves the reaction of 2-bromodibenzothiophene with ammonia or an amine under suitable conditions. Another method includes the use of phosphorus decasulfide or Lawesson’s reagent as sulfur-transferring agents to convert linear alkynyl nitriles into 2-aminothiophenes .
Industrial Production Methods: Industrial production of this compound typically involves large-scale synthesis using optimized reaction conditions to maximize yield and purity. The process often includes steps such as refluxing, distillation, and purification through recrystallization or chromatography .
Chemical Reactions Analysis
Types of Reactions: 2-Dibenzothiophenamine undergoes various chemical reactions, including:
Oxidation: It can be oxidized to form sulfoxides or sulfones.
Reduction: The compound can be reduced to form thiols or other reduced derivatives.
Substitution: Electrophilic substitution reactions can occur at the aromatic ring, leading to various substituted derivatives.
Common Reagents and Conditions:
Oxidation: Common oxidizing agents include hydrogen peroxide and peracids.
Reduction: Reducing agents such as lithium aluminum hydride or sodium borohydride are often used.
Substitution: Reagents like halogens, nitrating agents, and sulfonating agents are employed under controlled conditions.
Major Products Formed:
Oxidation: Sulfoxides and sulfones.
Reduction: Thiols and other reduced derivatives.
Substitution: Various substituted dibenzothiophenamine derivatives.
Scientific Research Applications
2-Dibenzothiophenamine has a wide range of applications in scientific research:
Chemistry: It is used as a building block in the synthesis of more complex heterocyclic compounds.
Biology: The compound is studied for its potential biological activities, including antimicrobial and antioxidant properties.
Medicine: Research is ongoing to explore its potential as a therapeutic agent in treating various diseases.
Industry: It is used in the development of materials with specific electronic and optical properties
Mechanism of Action
The mechanism of action of 2-Dibenzothiophenamine involves its interaction with specific molecular targets and pathways. It can act as an inhibitor or activator of certain enzymes, depending on its chemical structure and the nature of the target. The compound’s effects are mediated through binding to active sites or allosteric sites on proteins, leading to changes in their activity and function .
Comparison with Similar Compounds
- 2-Aminodibenzothiophene
- 2-Bromodibenzothiophene
- 2-Fluorodibenzothiophene
Comparison: 2-Dibenzothiophenamine is unique due to its specific amino substitution at the second carbon of the dibenzothiophene core. This substitution significantly influences its chemical reactivity and biological activity compared to other similar compounds. For instance, 2-bromodibenzothiophene is more reactive in substitution reactions due to the presence of a bromine atom, while 2-fluorodibenzothiophene exhibits different electronic properties due to the fluorine atom .
Properties
IUPAC Name |
dibenzothiophen-2-amine | |
---|---|---|
Source | PubChem | |
URL | https://pubchem.ncbi.nlm.nih.gov | |
Description | Data deposited in or computed by PubChem | |
InChI |
InChI=1S/C12H9NS/c13-8-5-6-12-10(7-8)9-3-1-2-4-11(9)14-12/h1-7H,13H2 | |
Source | PubChem | |
URL | https://pubchem.ncbi.nlm.nih.gov | |
Description | Data deposited in or computed by PubChem | |
InChI Key |
ICMMJWDNKMITSS-UHFFFAOYSA-N | |
Source | PubChem | |
URL | https://pubchem.ncbi.nlm.nih.gov | |
Description | Data deposited in or computed by PubChem | |
Canonical SMILES |
C1=CC=C2C(=C1)C3=C(S2)C=CC(=C3)N | |
Source | PubChem | |
URL | https://pubchem.ncbi.nlm.nih.gov | |
Description | Data deposited in or computed by PubChem | |
Molecular Formula |
C12H9NS | |
Source | PubChem | |
URL | https://pubchem.ncbi.nlm.nih.gov | |
Description | Data deposited in or computed by PubChem | |
DSSTOX Substance ID |
DTXSID50305375 | |
Record name | 2-Dibenzothiophenamine | |
Source | EPA DSSTox | |
URL | https://comptox.epa.gov/dashboard/DTXSID50305375 | |
Description | DSSTox provides a high quality public chemistry resource for supporting improved predictive toxicology. | |
Molecular Weight |
199.27 g/mol | |
Source | PubChem | |
URL | https://pubchem.ncbi.nlm.nih.gov | |
Description | Data deposited in or computed by PubChem | |
CAS No. |
7428-91-3 | |
Record name | 2-Dibenzothiophenamine | |
Source | DTP/NCI | |
URL | https://dtp.cancer.gov/dtpstandard/servlet/dwindex?searchtype=NSC&outputformat=html&searchlist=170576 | |
Description | The NCI Development Therapeutics Program (DTP) provides services and resources to the academic and private-sector research communities worldwide to facilitate the discovery and development of new cancer therapeutic agents. | |
Explanation | Unless otherwise indicated, all text within NCI products is free of copyright and may be reused without our permission. Credit the National Cancer Institute as the source. | |
Record name | 2-Dibenzothiophenamine | |
Source | EPA DSSTox | |
URL | https://comptox.epa.gov/dashboard/DTXSID50305375 | |
Description | DSSTox provides a high quality public chemistry resource for supporting improved predictive toxicology. | |
Record name | Dibenzo[b,d]thiophen-2-amine | |
Source | European Chemicals Agency (ECHA) | |
URL | https://echa.europa.eu/information-on-chemicals | |
Description | The European Chemicals Agency (ECHA) is an agency of the European Union which is the driving force among regulatory authorities in implementing the EU's groundbreaking chemicals legislation for the benefit of human health and the environment as well as for innovation and competitiveness. | |
Explanation | Use of the information, documents and data from the ECHA website is subject to the terms and conditions of this Legal Notice, and subject to other binding limitations provided for under applicable law, the information, documents and data made available on the ECHA website may be reproduced, distributed and/or used, totally or in part, for non-commercial purposes provided that ECHA is acknowledged as the source: "Source: European Chemicals Agency, http://echa.europa.eu/". Such acknowledgement must be included in each copy of the material. ECHA permits and encourages organisations and individuals to create links to the ECHA website under the following cumulative conditions: Links can only be made to webpages that provide a link to the Legal Notice page. | |
Retrosynthesis Analysis
AI-Powered Synthesis Planning: Our tool employs the Template_relevance Pistachio, Template_relevance Bkms_metabolic, Template_relevance Pistachio_ringbreaker, Template_relevance Reaxys, Template_relevance Reaxys_biocatalysis model, leveraging a vast database of chemical reactions to predict feasible synthetic routes.
One-Step Synthesis Focus: Specifically designed for one-step synthesis, it provides concise and direct routes for your target compounds, streamlining the synthesis process.
Accurate Predictions: Utilizing the extensive PISTACHIO, BKMS_METABOLIC, PISTACHIO_RINGBREAKER, REAXYS, REAXYS_BIOCATALYSIS database, our tool offers high-accuracy predictions, reflecting the latest in chemical research and data.
Strategy Settings
Precursor scoring | Relevance Heuristic |
---|---|
Min. plausibility | 0.01 |
Model | Template_relevance |
Template Set | Pistachio/Bkms_metabolic/Pistachio_ringbreaker/Reaxys/Reaxys_biocatalysis |
Top-N result to add to graph | 6 |
Feasible Synthetic Routes
Q2: What was the goal of synthesizing the []benzothieno[3,2-f]quinazoline-1,3-diamine derivative from 2-Dibenzothiophenamine?
A1: The researchers aimed to synthesize []benzothieno[3,2-f]quinazoline-1,3-diamine and its 7,7-dioxide derivative starting from 1-bromo-2-dibenzothiophenamine, which was derived from this compound []. The synthesized compounds were then tested for antimalarial activity.
Q3: Did the synthesized []benzothieno[3,2-f]quinazoline-1,3-diamine derivatives show any antimalarial activity?
A2: Unfortunately, both []benzothieno[3,2-f]quinazoline-1,3-diamine and its corresponding 7,7-dioxide derivative were found to be inactive against malaria []. This suggests that further structural modifications may be needed to obtain derivatives with desirable antimalarial properties.
Disclaimer and Information on In-Vitro Research Products
Please be aware that all articles and product information presented on BenchChem are intended solely for informational purposes. The products available for purchase on BenchChem are specifically designed for in-vitro studies, which are conducted outside of living organisms. In-vitro studies, derived from the Latin term "in glass," involve experiments performed in controlled laboratory settings using cells or tissues. It is important to note that these products are not categorized as medicines or drugs, and they have not received approval from the FDA for the prevention, treatment, or cure of any medical condition, ailment, or disease. We must emphasize that any form of bodily introduction of these products into humans or animals is strictly prohibited by law. It is essential to adhere to these guidelines to ensure compliance with legal and ethical standards in research and experimentation.