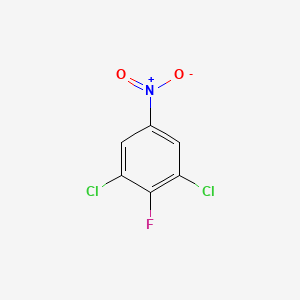
3,5-Dichloro-4-fluoronitrobenzene
Overview
Description
3,5-Dichloro-4-fluoronitrobenzene is an aromatic compound with the molecular formula C6H2Cl2FNO2 It is a light yellow to brown powder or crystalline substance
Preparation Methods
Synthetic Routes and Reaction Conditions
3,5-Dichloro-4-fluoronitrobenzene can be synthesized through a multi-step process involving the nitration of 1,3-dichloro-2-fluorobenzene. The nitration reaction typically involves the use of concentrated nitric acid and sulfuric acid as reagents. The reaction is carried out under controlled temperature conditions to ensure the selective introduction of the nitro group at the desired position on the benzene ring.
Industrial Production Methods
In industrial settings, the production of 1,3-dichloro-2-fluoro-5-nitrobenzene may involve large-scale nitration reactors with precise control over reaction parameters such as temperature, concentration, and reaction time. The product is then purified through crystallization or distillation to achieve the desired purity levels.
Chemical Reactions Analysis
Types of Reactions
3,5-Dichloro-4-fluoronitrobenzene undergoes various types of chemical reactions, including:
Nucleophilic Substitution: The chlorine atoms on the benzene ring can be replaced by nucleophiles such as amines or alkoxides.
Reduction: The nitro group can be reduced to an amino group using reducing agents like iron powder or catalytic hydrogenation.
Electrophilic Aromatic Substitution: The compound can undergo further substitution reactions with electrophiles, such as halogenation or sulfonation.
Common Reagents and Conditions
Nucleophilic Substitution: Reagents like sodium methoxide or ammonia in solvents such as methanol or ethanol.
Reduction: Iron powder in the presence of hydrochloric acid or catalytic hydrogenation using palladium on carbon.
Electrophilic Aromatic Substitution: Halogenation using chlorine or bromine in the presence of a Lewis acid catalyst like aluminum chloride.
Major Products Formed
Nucleophilic Substitution: Formation of substituted derivatives such as 1,3-dichloro-2-fluoro-5-aminobenzene.
Reduction: Formation of 1,3-dichloro-2-fluoro-5-aminobenzene.
Electrophilic Aromatic Substitution: Formation of halogenated or sulfonated derivatives.
Scientific Research Applications
3,5-Dichloro-4-fluoronitrobenzene has several applications in scientific research, including:
Chemistry: Used as an intermediate in the synthesis of various organic compounds, including pharmaceuticals and agrochemicals.
Biology: Studied for its potential biological activity and interactions with biomolecules.
Medicine: Investigated for its potential use in drug development and as a building block for active pharmaceutical ingredients.
Industry: Utilized in the production of specialty chemicals and materials with specific properties.
Mechanism of Action
The mechanism of action of 1,3-dichloro-2-fluoro-5-nitrobenzene involves its interactions with molecular targets through its functional groups. The nitro group can participate in redox reactions, while the halogen atoms can engage in nucleophilic substitution reactions. These interactions can lead to the formation of various derivatives with different biological and chemical properties.
Comparison with Similar Compounds
Similar Compounds
1,3-Dichloro-5-fluoro-2-nitrobenzene: Similar structure but with the nitro group in a different position.
1,2-Dichloro-4-fluoro-5-nitrobenzene: Similar structure with different positions of the chlorine and fluorine atoms.
1,3-Dichloro-5-nitrobenzene: Lacks the fluorine atom but has a similar arrangement of chlorine and nitro groups.
Uniqueness
3,5-Dichloro-4-fluoronitrobenzene is unique due to the specific arrangement of its functional groups, which imparts distinct chemical reactivity and potential applications. The presence of both chlorine and fluorine atoms, along with the nitro group, allows for a wide range of chemical transformations and interactions, making it a valuable compound in various fields of research and industry.
Properties
IUPAC Name |
1,3-dichloro-2-fluoro-5-nitrobenzene | |
---|---|---|
Source | PubChem | |
URL | https://pubchem.ncbi.nlm.nih.gov | |
Description | Data deposited in or computed by PubChem | |
InChI |
InChI=1S/C6H2Cl2FNO2/c7-4-1-3(10(11)12)2-5(8)6(4)9/h1-2H | |
Source | PubChem | |
URL | https://pubchem.ncbi.nlm.nih.gov | |
Description | Data deposited in or computed by PubChem | |
InChI Key |
VMAATSFMXSMKPG-UHFFFAOYSA-N | |
Source | PubChem | |
URL | https://pubchem.ncbi.nlm.nih.gov | |
Description | Data deposited in or computed by PubChem | |
Canonical SMILES |
C1=C(C=C(C(=C1Cl)F)Cl)[N+](=O)[O-] | |
Source | PubChem | |
URL | https://pubchem.ncbi.nlm.nih.gov | |
Description | Data deposited in or computed by PubChem | |
Molecular Formula |
C6H2Cl2FNO2 | |
Source | PubChem | |
URL | https://pubchem.ncbi.nlm.nih.gov | |
Description | Data deposited in or computed by PubChem | |
DSSTOX Substance ID |
DTXSID00185023 | |
Record name | 1,3-Dichloro-2-fluoro-5-nitrobenzene | |
Source | EPA DSSTox | |
URL | https://comptox.epa.gov/dashboard/DTXSID00185023 | |
Description | DSSTox provides a high quality public chemistry resource for supporting improved predictive toxicology. | |
Molecular Weight |
209.99 g/mol | |
Source | PubChem | |
URL | https://pubchem.ncbi.nlm.nih.gov | |
Description | Data deposited in or computed by PubChem | |
CAS No. |
3107-19-5 | |
Record name | 1,3-Dichloro-2-fluoro-5-nitrobenzene | |
Source | CAS Common Chemistry | |
URL | https://commonchemistry.cas.org/detail?cas_rn=3107-19-5 | |
Description | CAS Common Chemistry is an open community resource for accessing chemical information. Nearly 500,000 chemical substances from CAS REGISTRY cover areas of community interest, including common and frequently regulated chemicals, and those relevant to high school and undergraduate chemistry classes. This chemical information, curated by our expert scientists, is provided in alignment with our mission as a division of the American Chemical Society. | |
Explanation | The data from CAS Common Chemistry is provided under a CC-BY-NC 4.0 license, unless otherwise stated. | |
Record name | 1,3-Dichloro-2-fluoro-5-nitrobenzene | |
Source | ChemIDplus | |
URL | https://pubchem.ncbi.nlm.nih.gov/substance/?source=chemidplus&sourceid=0003107195 | |
Description | ChemIDplus is a free, web search system that provides access to the structure and nomenclature authority files used for the identification of chemical substances cited in National Library of Medicine (NLM) databases, including the TOXNET system. | |
Record name | 1,3-Dichloro-2-fluoro-5-nitrobenzene | |
Source | EPA DSSTox | |
URL | https://comptox.epa.gov/dashboard/DTXSID00185023 | |
Description | DSSTox provides a high quality public chemistry resource for supporting improved predictive toxicology. | |
Record name | 1,3-dichloro-2-fluoro-5-nitrobenzene | |
Source | European Chemicals Agency (ECHA) | |
URL | https://echa.europa.eu/substance-information/-/substanceinfo/100.019.515 | |
Description | The European Chemicals Agency (ECHA) is an agency of the European Union which is the driving force among regulatory authorities in implementing the EU's groundbreaking chemicals legislation for the benefit of human health and the environment as well as for innovation and competitiveness. | |
Explanation | Use of the information, documents and data from the ECHA website is subject to the terms and conditions of this Legal Notice, and subject to other binding limitations provided for under applicable law, the information, documents and data made available on the ECHA website may be reproduced, distributed and/or used, totally or in part, for non-commercial purposes provided that ECHA is acknowledged as the source: "Source: European Chemicals Agency, http://echa.europa.eu/". Such acknowledgement must be included in each copy of the material. ECHA permits and encourages organisations and individuals to create links to the ECHA website under the following cumulative conditions: Links can only be made to webpages that provide a link to the Legal Notice page. | |
Record name | 1,3-Dichloro-2-fluoro-5-nitrobenzene | |
Source | FDA Global Substance Registration System (GSRS) | |
URL | https://gsrs.ncats.nih.gov/ginas/app/beta/substances/7GS7VN6LFA | |
Description | The FDA Global Substance Registration System (GSRS) enables the efficient and accurate exchange of information on what substances are in regulated products. Instead of relying on names, which vary across regulatory domains, countries, and regions, the GSRS knowledge base makes it possible for substances to be defined by standardized, scientific descriptions. | |
Explanation | Unless otherwise noted, the contents of the FDA website (www.fda.gov), both text and graphics, are not copyrighted. They are in the public domain and may be republished, reprinted and otherwise used freely by anyone without the need to obtain permission from FDA. Credit to the U.S. Food and Drug Administration as the source is appreciated but not required. | |
Synthesis routes and methods
Procedure details
Retrosynthesis Analysis
AI-Powered Synthesis Planning: Our tool employs the Template_relevance Pistachio, Template_relevance Bkms_metabolic, Template_relevance Pistachio_ringbreaker, Template_relevance Reaxys, Template_relevance Reaxys_biocatalysis model, leveraging a vast database of chemical reactions to predict feasible synthetic routes.
One-Step Synthesis Focus: Specifically designed for one-step synthesis, it provides concise and direct routes for your target compounds, streamlining the synthesis process.
Accurate Predictions: Utilizing the extensive PISTACHIO, BKMS_METABOLIC, PISTACHIO_RINGBREAKER, REAXYS, REAXYS_BIOCATALYSIS database, our tool offers high-accuracy predictions, reflecting the latest in chemical research and data.
Strategy Settings
Precursor scoring | Relevance Heuristic |
---|---|
Min. plausibility | 0.01 |
Model | Template_relevance |
Template Set | Pistachio/Bkms_metabolic/Pistachio_ringbreaker/Reaxys/Reaxys_biocatalysis |
Top-N result to add to graph | 6 |
Feasible Synthetic Routes
Disclaimer and Information on In-Vitro Research Products
Please be aware that all articles and product information presented on BenchChem are intended solely for informational purposes. The products available for purchase on BenchChem are specifically designed for in-vitro studies, which are conducted outside of living organisms. In-vitro studies, derived from the Latin term "in glass," involve experiments performed in controlled laboratory settings using cells or tissues. It is important to note that these products are not categorized as medicines or drugs, and they have not received approval from the FDA for the prevention, treatment, or cure of any medical condition, ailment, or disease. We must emphasize that any form of bodily introduction of these products into humans or animals is strictly prohibited by law. It is essential to adhere to these guidelines to ensure compliance with legal and ethical standards in research and experimentation.