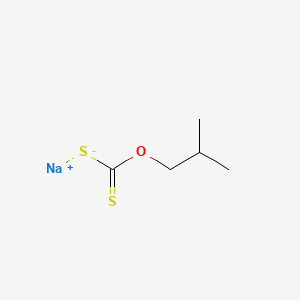
Sodium O-isobutyl dithiocarbonate
- Click on QUICK INQUIRY to receive a quote from our team of experts.
- With the quality product at a COMPETITIVE price, you can focus more on your research.
Overview
Description
Sodium O-isobutyl dithiocarbonate is a useful research compound. Its molecular formula is C5H10NaOS2 and its molecular weight is 173.3 g/mol. The purity is usually 95%.
BenchChem offers high-quality this compound suitable for many research applications. Different packaging options are available to accommodate customers' requirements. Please inquire for more information about this compound including the price, delivery time, and more detailed information at info@benchchem.com.
Mechanism of Action
Target of Action
Sodium Isobutyl Xanthate, also known as Iso-butyl xanthate, II or Sodium O-isobutyl dithiocarbonate, primarily targets sulphide minerals . It is used as a flotation reagent in the mining industry for the extraction of minerals such as copper, lead, and zinc . The compound’s effectiveness as a collector of sulphide ores is attributed to its unique structure .
Mode of Action
The mode of action of Sodium Isobutyl Xanthate involves its interaction with the sulphide mineral targets. It works by adsorbing onto the sulphide mineral surface and forming insoluble metal xanthates, which are highly hydrophobic . This hydrophobicity allows the mineral particles to attach to air bubbles and rise to the surface in a froth flotation process . The compound’s effectiveness in this process is determined by factors such as dosage and the particle size range of the minerals .
Biochemical Pathways
The biochemical pathways affected by Sodium Isobutyl Xanthate involve the conversion of hydrophilic mineral surfaces to hydrophobic ones. This is achieved through the formation of insoluble metal xanthates on the mineral surface . The compound’s interaction with its targets leads to changes in the surface properties of the minerals, enabling them to attach to air bubbles and be separated from the ore .
Result of Action
The result of Sodium Isobutyl Xanthate’s action is the successful separation of valuable minerals from ores. By rendering the mineral surfaces hydrophobic, the compound allows for the efficient recovery of minerals through froth flotation . The compound has been shown to achieve high recovery rates for various minerals at different dosages .
Action Environment
The action of Sodium Isobutyl Xanthate is influenced by environmental factors such as pH and temperature . The compound is known to be unstable under acidic and alkaline conditions, with its decomposition rate increasing with temperature and decreasing pH . Furthermore, the compound’s effectiveness in mineral extraction can be affected by the physical properties of the minerals, such as their particle size .
Biochemical Analysis
Biochemical Properties
Sodium O-isobutyl dithiocarbonate plays a significant role in biochemical reactions, particularly in the context of its interactions with metal ions. It acts as a chelating agent, forming stable complexes with transition metals such as copper, lead, and zinc . These interactions are crucial in various industrial applications, including mineral flotation and metal recovery processes. The compound’s ability to bind with metal ions is attributed to the presence of sulfur atoms in its structure, which have a high affinity for metal cations .
Cellular Effects
The effects of this compound on cellular processes are primarily related to its interactions with metal ions. In cells, this compound can influence metal homeostasis by binding to metal ions and altering their availability for cellular functions . This can impact various cellular processes, including enzyme activity, gene expression, and cell signaling pathways. For example, the binding of this compound to copper ions can inhibit the activity of copper-dependent enzymes, leading to changes in cellular metabolism and gene expression .
Molecular Mechanism
At the molecular level, this compound exerts its effects through the formation of metal complexes. The compound’s sulfur atoms coordinate with metal ions, forming stable chelates that can inhibit or activate specific enzymes . This chelation process can lead to enzyme inhibition by blocking the active sites of metalloenzymes or altering their conformation . Additionally, the binding of this compound to metal ions can affect the expression of genes involved in metal homeostasis and stress responses .
Temporal Effects in Laboratory Settings
In laboratory settings, the effects of this compound can change over time due to its stability and degradation properties. The compound is known to be hygroscopic, meaning it can absorb moisture from the environment, leading to its decomposition and the release of carbon disulfide . This degradation process can impact the long-term effects of the compound on cellular functions, as the availability of the active chelating agent decreases over time . In in vitro and in vivo studies, the stability of this compound is a critical factor in determining its efficacy and potential long-term effects .
Dosage Effects in Animal Models
The effects of this compound in animal models vary with different dosages. At low doses, the compound can effectively chelate metal ions and modulate their availability for biological processes . At high doses, this compound can exhibit toxic effects, including damage to organs and tissues due to the release of carbon disulfide during its degradation . Studies have shown that the compound can cause reproductive toxicity and other adverse effects at elevated dosages .
Metabolic Pathways
This compound is involved in metabolic pathways related to its chelation of metal ions. The compound can interact with enzymes and cofactors involved in metal metabolism, affecting the flux of metal ions within cells . For example, the chelation of copper ions by this compound can impact the activity of copper-dependent enzymes and alter the levels of copper-containing metabolites . Additionally, the degradation of the compound can lead to the formation of carbon disulfide, which can further influence metabolic processes .
Transport and Distribution
Within cells and tissues, this compound is transported and distributed based on its interactions with metal ions and transport proteins . The compound can bind to metal transporters and binding proteins, facilitating its movement across cellular membranes and its accumulation in specific tissues . The distribution of this compound is influenced by factors such as pH, temperature, and the presence of other metal ions .
Subcellular Localization
The subcellular localization of this compound is determined by its interactions with metal ions and cellular compartments . The compound can be directed to specific organelles, such as the mitochondria or lysosomes, where it can exert its chelating effects . Post-translational modifications and targeting signals can also influence the localization of this compound within cells . The presence of metal ions and the cellular environment play a crucial role in determining the compound’s subcellular distribution and activity .
Properties
CAS No. |
25306-75-6 |
---|---|
Molecular Formula |
C5H10NaOS2 |
Molecular Weight |
173.3 g/mol |
IUPAC Name |
sodium;2-methylpropoxymethanedithioate |
InChI |
InChI=1S/C5H10OS2.Na/c1-4(2)3-6-5(7)8;/h4H,3H2,1-2H3,(H,7,8); |
InChI Key |
VEXIJLUQHUGSOH-UHFFFAOYSA-N |
SMILES |
CC(C)COC(=S)[S-].[Na+] |
Canonical SMILES |
CC(C)COC(=S)S.[Na] |
Related CAS |
6791-12-4 (Parent) |
Origin of Product |
United States |
Retrosynthesis Analysis
AI-Powered Synthesis Planning: Our tool employs the Template_relevance Pistachio, Template_relevance Bkms_metabolic, Template_relevance Pistachio_ringbreaker, Template_relevance Reaxys, Template_relevance Reaxys_biocatalysis model, leveraging a vast database of chemical reactions to predict feasible synthetic routes.
One-Step Synthesis Focus: Specifically designed for one-step synthesis, it provides concise and direct routes for your target compounds, streamlining the synthesis process.
Accurate Predictions: Utilizing the extensive PISTACHIO, BKMS_METABOLIC, PISTACHIO_RINGBREAKER, REAXYS, REAXYS_BIOCATALYSIS database, our tool offers high-accuracy predictions, reflecting the latest in chemical research and data.
Strategy Settings
Precursor scoring | Relevance Heuristic |
---|---|
Min. plausibility | 0.01 |
Model | Template_relevance |
Template Set | Pistachio/Bkms_metabolic/Pistachio_ringbreaker/Reaxys/Reaxys_biocatalysis |
Top-N result to add to graph | 6 |
Feasible Synthetic Routes
A: SIBX functions by selectively adsorbing onto the surface of sulfide minerals like chalcopyrite, galena, and sphalerite. [, , , , , , , , ] This adsorption occurs due to the strong affinity of the dithiocarbonate group (-C(=S)S-) for metal cations present on the mineral surface, rendering the mineral hydrophobic. [, , , , ] Air bubbles introduced into the flotation pulp then attach to the hydrophobized minerals, carrying them to the surface for collection as a concentrate. [, , , , , , , ]
A: Yes, the flotation performance of SIBX can be significantly influenced by the presence of other reagents. For instance, studies have shown that the use of copper sulfate as an activator can negatively impact the recovery of platinum group minerals (PGMs) when SIBX is used as the collector. [] Additionally, the presence of tetrathionate ions, often found in recycled process waters, can react with and consume SIBX, potentially affecting its dosage and flotation efficiency. []
ANone: The molecular formula of SIBX is C5H9OS2Na, and its molecular weight is 176.25 g/mol.
A: Various spectroscopic techniques have been employed to characterize SIBX, including infrared (IR) spectroscopy, 1H and 13C nuclear magnetic resonance (NMR) spectroscopy, and X-ray photoelectron spectroscopy (XPS). [, , ] IR spectroscopy helps identify functional groups, NMR provides insights into the compound's structure, and XPS examines the elemental composition and chemical states of the adsorbed SIBX on mineral surfaces.
A: The effectiveness of SIBX can be influenced by the pH of the flotation pulp. Research suggests that SIBX might exhibit optimal performance within a specific pH range, and its collecting ability could be altered at extremely acidic or alkaline conditions. [] It's crucial to optimize the pH for each specific flotation system to ensure maximum recovery and selectivity.
A: Yes, several alternatives to SIBX exist, including other xanthates, dithiophosphates, and thionocarbamates. [, , ] The choice of collector often depends on the specific minerals being targeted, the ore composition, and desired flotation selectivity. For instance, dithiophosphates have been explored for their potential to enhance copper recovery from a new copper ore deposit in Norway. []
A: The widespread use of SIBX in froth flotation raises concerns about its potential environmental impact. Xanthates, including SIBX, are known to release carbon disulfide (CS2) during their usage, contributing to air pollution. [] Additionally, residual xanthates in wastewater streams can pose risks to aquatic ecosystems.
A: Researchers are actively exploring strategies to minimize the environmental footprint of SIBX. One approach involves developing alternative collectors with potentially less harmful degradation products. [] Another area of focus is improving the treatment of xanthate-containing wastewater. For instance, flocculation processes using aluminum sulfate (Al2(SO4)3) have shown promise in effectively removing SIBX from wastewater. []
A: Effective SIBX research relies on a combination of experimental techniques and computational tools. Key experimental techniques include microflotation tests, zeta potential measurements, adsorption studies, and surface analysis methods like FTIR and XPS. [, , , ] These techniques provide valuable insights into SIBX's interaction with mineral surfaces and its behavior in flotation systems. On the computational front, density functional theory (DFT) calculations have emerged as a powerful tool to elucidate the adsorption mechanisms of SIBX and its structural analogs on mineral surfaces. []
A: The development and widespread adoption of xanthates, including SIBX, as flotation collectors marked a significant milestone in mineral processing. Early research focused on understanding the fundamental interactions between xanthates and sulfide minerals, paving the way for optimizing flotation processes. [, ] More recent research efforts have explored the use of modified xanthate structures, such as S-benzoyl O-isobutyl xanthate (BIBX), to enhance flotation selectivity for specific minerals like chalcopyrite. []
Disclaimer and Information on In-Vitro Research Products
Please be aware that all articles and product information presented on BenchChem are intended solely for informational purposes. The products available for purchase on BenchChem are specifically designed for in-vitro studies, which are conducted outside of living organisms. In-vitro studies, derived from the Latin term "in glass," involve experiments performed in controlled laboratory settings using cells or tissues. It is important to note that these products are not categorized as medicines or drugs, and they have not received approval from the FDA for the prevention, treatment, or cure of any medical condition, ailment, or disease. We must emphasize that any form of bodily introduction of these products into humans or animals is strictly prohibited by law. It is essential to adhere to these guidelines to ensure compliance with legal and ethical standards in research and experimentation.