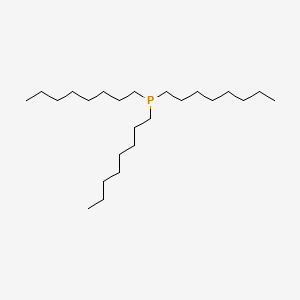
Trioctylphosphine
Overview
Description
Trioctylphosphine (TOP) is an organophosphorus compound with the formula P(C8H17)3 . It is usually encountered as a syrup and is colorless .
Synthesis Analysis
Trioctylphosphine has been used in the synthesis of metal nanoparticles . It has been used as both solvent and stabilizer to synthesize CdS nanorods . When Pd nanoparticles react with a TOP-Te complex, PdTe forms . This result demonstrates that the metal telluride phase that forms can be controlled by the reactivity of the tellurium reagent .Molecular Structure Analysis
The linear formula of Trioctylphosphine is [CH3(CH2)7]3P . The molecular weight is 370.64 .Chemical Reactions Analysis
Trioctylphosphine reacts with oxygen to form trioctylphosphine oxide . For this reason, it is usually handled with air-free techniques . TOP also reacts with elemental selenium to give trioctylphosphine selenide (TOPSe), which is a reagent for the preparation of cadmium selenide and related semiconductors .Physical And Chemical Properties Analysis
Trioctylphosphine has a molar mass of 370.635498 and a density of 0.831 g/mL . Its boiling point is 284 to 291 °C at 50 mmHg .Scientific Research Applications
Conversion to Metal Phosphides
Trioctylphosphine is utilized for converting various metal forms such as nanocrystals, bulk powders, foils, wires, and thin films into metal phosphides. This process is crucial in materials science for creating compounds with specific electronic and structural properties .
Synthesis of Cadmium Sulfide Nanorods
It acts as both a solvent and a stabilizer in the synthesis of cadmium sulfide nanorods from cadmium acetate and sulfur. These nanorods have significant applications in optoelectronics and photovoltaics .
Coating Quantum Dots
This compound is used in coating zinc sulfide shells on cadmium-selenium quantum dot cores, a method known as successive ionic layer adsorption and reaction (SILAR). Quantum dots have applications in display technologies and biological imaging .
Precursor to Trioctylphosphine Oxide
Trioctylphosphine serves as a precursor to trioctylphosphine oxide (TOPO), which is widely used in nanoparticle synthesis and as an extraction agent in hydrometallurgy .
Solvent for Metal Precursors
It is employed as a solvent for cadmium and selenium precursors in various chemical reactions, facilitating the synthesis of compounds under controlled conditions .
Low-Temperature Synthesis of Metal Phosphides
Trioctylphosphine enables the low-temperature synthesis of metal phosphides, which is an alternative approach that allows for the creation of these materials at temperatures below 370°C. This method is beneficial for producing phosphides with minimal thermal degradation .
Role in Colloidal and Emission Properties
Research has been conducted on trioctylphosphine’s role in colloidal synthesis and emission properties of certain perovskite nanocrystals, contributing to the understanding of their stability and optical features .
Mechanism of Action
Safety and Hazards
properties
IUPAC Name |
trioctylphosphane | |
---|---|---|
Source | PubChem | |
URL | https://pubchem.ncbi.nlm.nih.gov | |
Description | Data deposited in or computed by PubChem | |
InChI |
InChI=1S/C24H51P/c1-4-7-10-13-16-19-22-25(23-20-17-14-11-8-5-2)24-21-18-15-12-9-6-3/h4-24H2,1-3H3 | |
Source | PubChem | |
URL | https://pubchem.ncbi.nlm.nih.gov | |
Description | Data deposited in or computed by PubChem | |
InChI Key |
RMZAYIKUYWXQPB-UHFFFAOYSA-N | |
Source | PubChem | |
URL | https://pubchem.ncbi.nlm.nih.gov | |
Description | Data deposited in or computed by PubChem | |
Canonical SMILES |
CCCCCCCCP(CCCCCCCC)CCCCCCCC | |
Source | PubChem | |
URL | https://pubchem.ncbi.nlm.nih.gov | |
Description | Data deposited in or computed by PubChem | |
Molecular Formula |
C24H51P | |
Source | PubChem | |
URL | https://pubchem.ncbi.nlm.nih.gov | |
Description | Data deposited in or computed by PubChem | |
DSSTOX Substance ID |
DTXSID9063582 | |
Record name | Phosphine, trioctyl- | |
Source | EPA DSSTox | |
URL | https://comptox.epa.gov/dashboard/DTXSID9063582 | |
Description | DSSTox provides a high quality public chemistry resource for supporting improved predictive toxicology. | |
Molecular Weight |
370.6 g/mol | |
Source | PubChem | |
URL | https://pubchem.ncbi.nlm.nih.gov | |
Description | Data deposited in or computed by PubChem | |
Physical Description |
Liquid, Colorless liquid with an odor like garlic; [Alfa Aesar MSDS] | |
Record name | Phosphine, trioctyl- | |
Source | EPA Chemicals under the TSCA | |
URL | https://www.epa.gov/chemicals-under-tsca | |
Description | EPA Chemicals under the Toxic Substances Control Act (TSCA) collection contains information on chemicals and their regulations under TSCA, including non-confidential content from the TSCA Chemical Substance Inventory and Chemical Data Reporting. | |
Record name | Trioctylphosphine | |
Source | Haz-Map, Information on Hazardous Chemicals and Occupational Diseases | |
URL | https://haz-map.com/Agents/18394 | |
Description | Haz-Map® is an occupational health database designed for health and safety professionals and for consumers seeking information about the adverse effects of workplace exposures to chemical and biological agents. | |
Explanation | Copyright (c) 2022 Haz-Map(R). All rights reserved. Unless otherwise indicated, all materials from Haz-Map are copyrighted by Haz-Map(R). No part of these materials, either text or image may be used for any purpose other than for personal use. Therefore, reproduction, modification, storage in a retrieval system or retransmission, in any form or by any means, electronic, mechanical or otherwise, for reasons other than personal use, is strictly prohibited without prior written permission. | |
Product Name |
Trioctylphosphine | |
CAS RN |
4731-53-7 | |
Record name | Trioctylphosphine | |
Source | CAS Common Chemistry | |
URL | https://commonchemistry.cas.org/detail?cas_rn=4731-53-7 | |
Description | CAS Common Chemistry is an open community resource for accessing chemical information. Nearly 500,000 chemical substances from CAS REGISTRY cover areas of community interest, including common and frequently regulated chemicals, and those relevant to high school and undergraduate chemistry classes. This chemical information, curated by our expert scientists, is provided in alignment with our mission as a division of the American Chemical Society. | |
Explanation | The data from CAS Common Chemistry is provided under a CC-BY-NC 4.0 license, unless otherwise stated. | |
Record name | Trioctylphosphine | |
Source | ChemIDplus | |
URL | https://pubchem.ncbi.nlm.nih.gov/substance/?source=chemidplus&sourceid=0004731537 | |
Description | ChemIDplus is a free, web search system that provides access to the structure and nomenclature authority files used for the identification of chemical substances cited in National Library of Medicine (NLM) databases, including the TOXNET system. | |
Record name | TRIOCTYLPHOSPHINE | |
Source | DTP/NCI | |
URL | https://dtp.cancer.gov/dtpstandard/servlet/dwindex?searchtype=NSC&outputformat=html&searchlist=102529 | |
Description | The NCI Development Therapeutics Program (DTP) provides services and resources to the academic and private-sector research communities worldwide to facilitate the discovery and development of new cancer therapeutic agents. | |
Explanation | Unless otherwise indicated, all text within NCI products is free of copyright and may be reused without our permission. Credit the National Cancer Institute as the source. | |
Record name | Phosphine, trioctyl- | |
Source | EPA Chemicals under the TSCA | |
URL | https://www.epa.gov/chemicals-under-tsca | |
Description | EPA Chemicals under the Toxic Substances Control Act (TSCA) collection contains information on chemicals and their regulations under TSCA, including non-confidential content from the TSCA Chemical Substance Inventory and Chemical Data Reporting. | |
Record name | Phosphine, trioctyl- | |
Source | EPA DSSTox | |
URL | https://comptox.epa.gov/dashboard/DTXSID9063582 | |
Description | DSSTox provides a high quality public chemistry resource for supporting improved predictive toxicology. | |
Record name | Trioctylphosphine | |
Source | European Chemicals Agency (ECHA) | |
URL | https://echa.europa.eu/substance-information/-/substanceinfo/100.022.940 | |
Description | The European Chemicals Agency (ECHA) is an agency of the European Union which is the driving force among regulatory authorities in implementing the EU's groundbreaking chemicals legislation for the benefit of human health and the environment as well as for innovation and competitiveness. | |
Explanation | Use of the information, documents and data from the ECHA website is subject to the terms and conditions of this Legal Notice, and subject to other binding limitations provided for under applicable law, the information, documents and data made available on the ECHA website may be reproduced, distributed and/or used, totally or in part, for non-commercial purposes provided that ECHA is acknowledged as the source: "Source: European Chemicals Agency, http://echa.europa.eu/". Such acknowledgement must be included in each copy of the material. ECHA permits and encourages organisations and individuals to create links to the ECHA website under the following cumulative conditions: Links can only be made to webpages that provide a link to the Legal Notice page. | |
Record name | TRIOCTYLPHOSPHINE | |
Source | FDA Global Substance Registration System (GSRS) | |
URL | https://gsrs.ncats.nih.gov/ginas/app/beta/substances/VE0Y037UCN | |
Description | The FDA Global Substance Registration System (GSRS) enables the efficient and accurate exchange of information on what substances are in regulated products. Instead of relying on names, which vary across regulatory domains, countries, and regions, the GSRS knowledge base makes it possible for substances to be defined by standardized, scientific descriptions. | |
Explanation | Unless otherwise noted, the contents of the FDA website (www.fda.gov), both text and graphics, are not copyrighted. They are in the public domain and may be republished, reprinted and otherwise used freely by anyone without the need to obtain permission from FDA. Credit to the U.S. Food and Drug Administration as the source is appreciated but not required. | |
Synthesis routes and methods I
Procedure details
Synthesis routes and methods II
Procedure details
Retrosynthesis Analysis
AI-Powered Synthesis Planning: Our tool employs the Template_relevance Pistachio, Template_relevance Bkms_metabolic, Template_relevance Pistachio_ringbreaker, Template_relevance Reaxys, Template_relevance Reaxys_biocatalysis model, leveraging a vast database of chemical reactions to predict feasible synthetic routes.
One-Step Synthesis Focus: Specifically designed for one-step synthesis, it provides concise and direct routes for your target compounds, streamlining the synthesis process.
Accurate Predictions: Utilizing the extensive PISTACHIO, BKMS_METABOLIC, PISTACHIO_RINGBREAKER, REAXYS, REAXYS_BIOCATALYSIS database, our tool offers high-accuracy predictions, reflecting the latest in chemical research and data.
Strategy Settings
Precursor scoring | Relevance Heuristic |
---|---|
Min. plausibility | 0.01 |
Model | Template_relevance |
Template Set | Pistachio/Bkms_metabolic/Pistachio_ringbreaker/Reaxys/Reaxys_biocatalysis |
Top-N result to add to graph | 6 |
Feasible Synthetic Routes
Q & A
Q1: How does trioctylphosphine interact with cadmium selenide (CdSe) quantum dots?
A1: [, , , , , , , ] Trioctylphosphine interacts with CdSe quantum dots primarily through its phosphorus atom, which coordinates to selenium atoms on the quantum dot surface. This interaction leads to the formation of trioctylphosphine selenide (TOPSe) species on the surface. Trioctylphosphine oxide (TOPO), often present alongside TOP, coordinates to cadmium atoms on the surface. These interactions influence the surface passivation, photoluminescence efficiency, and stability of the quantum dots.
Q2: What is the role of trioctylphosphine in the synthesis of metal phosphide nanocrystals?
A2: [] Trioctylphosphine serves as both a phosphorus source and a stabilizing ligand in the synthesis of metal phosphide nanocrystals. At elevated temperatures, it decomposes to provide phosphorus, which reacts with metal precursors to form the desired metal phosphide phase. The remaining trioctylphosphine molecules then coordinate to the surface of the forming nanocrystals, providing steric stabilization and preventing aggregation.
Q3: What is the molecular formula and weight of trioctylphosphine?
A3: The molecular formula of trioctylphosphine is C24H51P. Its molecular weight is 366.65 g/mol.
Q4: Are there any spectroscopic techniques used to characterize trioctylphosphine?
A4: [, , ] Yes, 31P nuclear magnetic resonance (NMR) spectroscopy is commonly employed to characterize trioctylphosphine and its interactions with other compounds. The chemical shift of the phosphorus atom in 31P NMR provides information about the chemical environment and bonding of trioctylphosphine.
Q5: How does trioctylphosphine behave in high-boiling solvents?
A5: [, ] Trioctylphosphine is often used in conjunction with high-boiling solvents like trioctylphosphine oxide and oleylamine during nanoparticle synthesis. Its role can be influenced by the specific solvent used. For example, in the synthesis of nickel phosphide, the use of trioctylphosphine oxide as a coordinating solvent led to teardrop-shaped particles, while oleylamine resulted in less uniform shapes. []
Q6: Have computational methods been used to study trioctylphosphine?
A8: [] Yes, computational chemistry, particularly density functional theory (DFT) calculations, has been employed to investigate the interactions of trioctylphosphine with materials like cadmium sulfide (CdS) nanoparticles. [] These calculations help elucidate how trioctylphosphine influences the surface energy and ultimately the preferred crystal structure of the nanoparticles.
Q7: How do structural modifications of trioctylphosphine affect its properties?
A7: While the provided research focuses primarily on trioctylphosphine, it highlights the importance of the phosphine group for its interactions. Structural modifications, particularly alterations to the alkyl chains, could influence its steric hindrance and thus its ability to coordinate to surfaces and stabilize nanocrystals. Further research would be needed to explore specific structure-activity relationships.
Q8: Is trioctylphosphine stable under ambient conditions?
A8: Trioctylphosphine is generally stable under ambient conditions, but it can be prone to oxidation over time. Storage under inert gas is often recommended to maintain its purity.
Q9: Are there specific safety and environmental considerations when working with trioctylphosphine?
A9: While not extensively covered in the provided research, trioctylphosphine, like many organophosphorus compounds, requires careful handling. It's essential to consult safety data sheets and adhere to recommended laboratory practices. Research into its environmental impact and degradation pathways would be beneficial for responsible waste management and potential substitutes.
Q10: How are thin films of quantum dots containing trioctylphosphine characterized?
A12: [] Thin films of quantum dots prepared with trioctylphosphine are characterized using various techniques, including:
Q11: What techniques are used to study the interaction of trioctylphosphine with metal ions in solution?
A13: [] Spectrometric methods, particularly UV-Vis spectroscopy, are employed to study the interaction of trioctylphosphine with metal ions in solution. Changes in the absorption spectra provide information about complex formation, stoichiometry, and the nature of the interaction.
Disclaimer and Information on In-Vitro Research Products
Please be aware that all articles and product information presented on BenchChem are intended solely for informational purposes. The products available for purchase on BenchChem are specifically designed for in-vitro studies, which are conducted outside of living organisms. In-vitro studies, derived from the Latin term "in glass," involve experiments performed in controlled laboratory settings using cells or tissues. It is important to note that these products are not categorized as medicines or drugs, and they have not received approval from the FDA for the prevention, treatment, or cure of any medical condition, ailment, or disease. We must emphasize that any form of bodily introduction of these products into humans or animals is strictly prohibited by law. It is essential to adhere to these guidelines to ensure compliance with legal and ethical standards in research and experimentation.