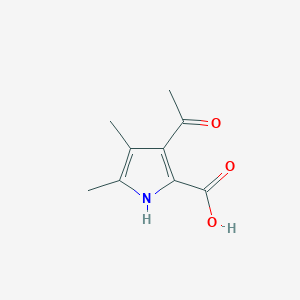
3-Acetyl-4,5-dimethyl-1H-pyrrole-2-carboxylic acid
Overview
Description
3-Acetyl-4,5-dimethyl-1H-pyrrole-2-carboxylic acid (CAS RN: 2386-33-6) is a heterocyclic compound with the molecular formula C₉H₁₁NO₃ and a molecular mass of 181.19 g/mol. Its structure features a pyrrole ring substituted with acetyl, methyl, and carboxylic acid groups (Figure 1). Key properties include a melting point of 204°C and solubility in polar solvents like ethanol and dichloromethane (DCM) . Industrially, it is used as a building block in pharmaceuticals and agrochemicals, with commercial availability at ≥95% purity .
Preparation Methods
Direct Acetylation of 4,5-Dimethyl-1H-pyrrole-2-carboxylic acid
Method Overview:
The most straightforward and commonly reported synthetic route involves the acetylation of 4,5-dimethyl-1H-pyrrole-2-carboxylic acid using acetic anhydride as the acetylating agent. This reaction is typically catalyzed by a base such as pyridine, which acts both as a catalyst and an acid scavenger.
- Starting Material: 4,5-dimethyl-1H-pyrrole-2-carboxylic acid
- Acetylating Agent: Acetic anhydride
- Catalyst: Pyridine
- Temperature: Elevated temperatures (typically reflux conditions) to facilitate acetylation
- Solvent: Often pyridine itself or an inert organic solvent compatible with the reagents
Process Description:
The reaction proceeds via nucleophilic attack of the pyrrole nitrogen or the activated position on the pyrrole ring by the acetyl group from acetic anhydride, resulting in the introduction of the acetyl substituent at the 3-position of the pyrrole ring. The carboxylic acid group remains intact during this process.
Purification:
Post-reaction, purification is achieved by recrystallization or chromatographic techniques to isolate the pure 3-acetyl derivative. These steps ensure removal of unreacted starting materials, pyridine, and acetic acid by-products.
- Simple one-step acetylation
- Readily available starting materials
- High selectivity for the 3-position acetylation
- Requires careful control of temperature and stoichiometry to avoid over-acetylation or side reactions
- Use of pyridine necessitates proper handling due to toxicity and odor
Multi-step Synthesis via Pyrrole Ring Construction and Functionalization
Method Overview:
An alternative approach involves constructing the pyrrole ring with the desired substitution pattern through multi-step reactions starting from simpler precursors, followed by selective functional group transformations.
- Bromination of Propionaldehyde: Propionaldehyde undergoes bromination in an aprotic solvent at 0–50 °C to form 2-bromopropanal.
- Ring Closure Reaction: 2-bromopropanal reacts with methyl acetoacetate and ammonia under alkaline conditions at 0–50 °C to form 2,4-dimethyl-1H-pyrrole-3-carboxylic acid ethyl ester or related derivatives.
- Subsequent Functionalization: The ester group can be hydrolyzed to the acid, and acetylation can be performed to install the acetyl group at the 3-position.
Reaction Conditions and Solvents:
- Bromination uses aprotic solvents such as toluene, methylene chloride, dimethylformamide, or dimethyl sulfoxide.
- Ring closure is performed under mild alkaline conditions using ammoniacal liquor.
- Allows precise control over substitution pattern on the pyrrole ring
- Suitable for large-scale industrial synthesis due to mild reaction conditions and accessible raw materials
- Avoids the use of expensive or environmentally harmful reagents like sodium nitrite or tert-butyl acetoacetate
- Multi-step process requiring intermediate purification
- Hydrolysis and acetylation steps demand careful control to maintain yield and purity
Industrial Production Considerations
In industrial settings, the synthesis of 3-acetyl-4,5-dimethyl-1H-pyrrole-2-carboxylic acid is optimized for yield, purity, and scalability. Batch or continuous flow processes are employed with advanced purification methods such as chromatography and recrystallization.
- Reaction parameters (temperature, catalyst concentration, reagent ratios) are finely tuned to maximize conversion.
- Use of environmentally benign solvents and reagents is preferred to minimize waste and toxicity.
- Process design includes steps for efficient removal of by-products and recovery of solvents.
Comparative Summary Table of Preparation Methods
Preparation Method | Starting Materials | Key Reagents & Conditions | Advantages | Limitations |
---|---|---|---|---|
Direct Acetylation | 4,5-dimethyl-1H-pyrrole-2-carboxylic acid | Acetic anhydride, pyridine, heat | Simple, selective, one-step | Requires careful control, pyridine handling |
Multi-step Synthesis via Bromination & Ring Closure | Propionaldehyde, methyl acetoacetate, ammonia | Bromination (Br2, aprotic solvent), ring closure (alkaline, 0–50 °C) | Mild conditions, scalable, avoids toxic reagents | Multi-step, intermediate purification needed |
Industrial Batch/Continuous Flow Processes | Same as above, optimized for scale | Optimized reaction parameters, advanced purification | High yield, purity, scalable | Requires sophisticated process control |
Research Findings and Notes
- The acetylation reaction mechanism involves nucleophilic attack on acetic anhydride facilitated by pyridine, leading to selective acetylation at the 3-position of the pyrrole ring.
- The presence of the carboxylic acid group enhances the compound's reactivity and potential for further derivatization, such as amide or ester formation.
- Industrial synthesis methods focus on environmentally friendly and cost-effective reagents, avoiding hazardous materials like sodium nitrite and expensive tert-butyl acetoacetate.
- The multi-step synthetic route via bromination and ring closure is preferred for large-scale production due to milder conditions and better control over product purity and yield.
Chemical Reactions Analysis
Types of Reactions: 3-Acetyl-4,5-dimethyl-1H-pyrrole-2-carboxylic acid undergoes various chemical reactions, including:
Oxidation: The compound can be oxidized to form corresponding pyrrole derivatives with higher oxidation states.
Reduction: Reduction reactions can convert the acetyl group to an alcohol or other reduced forms.
Substitution: Electrophilic and nucleophilic substitution reactions can occur at the pyrrole ring, leading to the formation of various substituted derivatives.
Common Reagents and Conditions:
Oxidation: Common oxidizing agents include potassium permanganate and chromium trioxide.
Reduction: Reducing agents such as sodium borohydride and lithium aluminum hydride are used.
Substitution: Reagents like halogens, alkyl halides, and nucleophiles are employed under appropriate conditions.
Major Products: The major products formed from these reactions depend on the specific reagents and conditions used. For example, oxidation may yield pyrrole-2,3-dicarboxylic acid derivatives, while reduction can produce alcohols or amines.
Scientific Research Applications
Chemistry
3-Acetyl-4,5-dimethyl-1H-pyrrole-2-carboxylic acid serves as an intermediate in synthesizing more complex heterocyclic compounds. Its unique structure allows for various chemical transformations, including:
- Oxidation: Can be oxidized to form corresponding carboxylic acids.
- Reduction: Reduction reactions can convert the carboxylic acid to alcohols.
- Substitution Reactions: Electrophilic substitution can occur at the pyrrole ring.
Research has highlighted several biological activities associated with this compound:
Antimicrobial Properties:
Studies have demonstrated significant antimicrobial activity against various bacterial strains such as Staphylococcus aureus and Escherichia coli. The mechanism of action often involves disrupting microbial cell membranes and inhibiting metabolic pathways.
Case Study:
A study published in a peer-reviewed journal evaluated the antimicrobial efficacy of this compound against several pathogens, showing notable inhibition zones compared to control substances .
Anticancer Potential:
Preliminary studies suggest that this compound may exhibit anticancer properties by inducing apoptosis in cancer cell lines. Further research is needed to elucidate the underlying mechanisms.
Pharmaceutical Development
The compound is being explored as a lead molecule for the development of new pharmaceuticals. Its structural characteristics make it a candidate for modifications aimed at enhancing biological activity and reducing toxicity.
Mechanism of Action
The mechanism of action of 3-Acetyl-4,5-dimethyl-1H-pyrrole-2-carboxylic acid involves its interaction with specific molecular targets and pathways. The acetyl group and the pyrrole ring play crucial roles in its reactivity and binding affinity. The compound can interact with enzymes, receptors, and other biomolecules, leading to various biological effects. Detailed studies are required to elucidate the exact molecular mechanisms and pathways involved.
Comparison with Similar Compounds
Comparison with Structurally Similar Compounds
Positional Isomers: 4-Acetyl-3,5-dimethyl-1H-pyrrole-2-carboxylic Acid
- Structural Difference : The acetyl group is located at the 4-position (CAS RN: 2386-28-9) instead of the 3-position.
- Physical Properties :
- Synthesis : Both isomers are synthesized via condensation reactions, but yields and purification steps may differ due to steric and electronic effects of substituent positioning.
Derivatives with Ester Groups: 4,5-Dimethyl-1H-pyrrole-2-carboxylic Acid Ethyl Ester
- Structural Difference: The carboxylic acid group is replaced by an ethyl ester (C₈H₁₁NO₂).
- Bioactivity : Found in marine seaweed (Asparagopsis taxiformis), this derivative exhibits antimicrobial activity against Leptospira javanica (MIC: 100–1600 µg/mL), though less potent than penicillin .
- Applications : Ester derivatives are often more lipophilic, enhancing membrane permeability in drug design .
Pyrrolopyridine Analogues: 1H-Pyrrolo[2,3-c]pyridine-2-carboxylic Acids
- Structural Difference : A fused pyridine ring replaces one pyrrole ring carbon, creating a bicyclic system (e.g., 5-chloro and 5-methoxy derivatives) .
- Synthesis Efficiency : High yields (71–95% ) via NaOH-mediated hydrolysis under reflux, indicating robust synthetic routes .
- Applications : These analogues are explored in medicinal chemistry for kinase inhibition, leveraging the pyridine ring’s hydrogen-bonding capacity .
Reduced-Ring Derivatives: 1-Acetyl-4,5-dihydro-1H-pyrrole-2-carboxylic Acid
- Structural Difference: The pyrrole ring is partially saturated (C₇H₉NO₃), reducing aromaticity .
- Physical Properties : Lower molecular mass (155.15 g/mol ) and altered solubility due to reduced planarity.
- Applications : Saturation may enhance stability in aqueous environments, useful for prodrug development .
Comparative Data Table
Key Research Findings
- Synthetic Flexibility : The pyrrole-carboxylic acid scaffold supports diverse modifications, including esterification, halogenation, and ring fusion, enabling tailored physicochemical properties .
- Bioactivity Correlations : Ester derivatives (e.g., ethyl ester) show moderate antimicrobial activity, while carboxylic acid forms are preferred for metal coordination in catalysis .
- Isomer-Specific Properties : Positional isomerism (3-acetyl vs. 4-acetyl) minimally affects melting points but may influence reactivity in coupling reactions .
Biological Activity
3-Acetyl-4,5-dimethyl-1H-pyrrole-2-carboxylic acid (ADMPCA) is a compound of significant interest in medicinal chemistry due to its diverse biological activities, particularly its antimicrobial and anticancer properties. This article provides a comprehensive overview of the biological activity associated with ADMPCA, including its mechanisms of action, relevant case studies, and research findings.
Chemical Structure and Properties
ADMPCA possesses a pyrrole ring structure with acetyl and dimethyl substituents. Its chemical formula is CHNO, and it can be synthesized through various methods, including acylation reactions involving pyrrole derivatives. The unique structure contributes to its reactivity and biological interactions.
The biological activity of ADMPCA is primarily attributed to its ability to interact with specific molecular targets, including enzymes and receptors. The acetyl group enhances the compound's lipophilicity, facilitating cellular uptake and interaction with biological membranes. Research suggests that ADMPCA modulates enzyme activity, which is crucial for its antimicrobial and anticancer effects .
Antimicrobial Activity
ADMPCA has shown promising antimicrobial properties against various pathogens. In vitro studies indicate that it exhibits activity against both Gram-positive and Gram-negative bacteria. The Minimum Inhibitory Concentration (MIC) values for several bacterial strains are summarized in the following table:
Pathogen | MIC (µg/mL) |
---|---|
Staphylococcus aureus | 32 |
Escherichia coli | 64 |
Klebsiella pneumoniae | 64 |
Pseudomonas aeruginosa | 128 |
These results suggest that while ADMPCA is effective against certain pathogens, its activity can vary significantly depending on the bacterial strain .
Anticancer Activity
Research has also explored the anticancer potential of ADMPCA, particularly in human lung adenocarcinoma (A549) models. In a comparative study using an MTT assay, ADMPCA demonstrated a reduction in cell viability at concentrations around 100 µM. The results are illustrated in the following table:
Compound | Post-Treatment Viability (%) |
---|---|
ADMPCA (100 µM) | 78 |
Cisplatin (Control) | 66 |
Untreated Control | 100 |
While ADMPCA showed moderate cytotoxicity, it was less effective than cisplatin, a standard chemotherapeutic agent .
Case Studies
- Antimicrobial Efficacy : A study evaluated the antimicrobial activity of ADMPCA derivatives against multidrug-resistant strains of bacteria. The results indicated that modifications to the pyrrole structure could enhance antibacterial efficacy, suggesting a pathway for developing more potent derivatives .
- Cytotoxicity in Cancer Cells : Another investigation focused on the cytotoxic effects of ADMPCA on A549 cells compared to non-cancerous human small airway epithelial cells (HSAEC-1 KT). The findings revealed that while ADMPCA affected cancer cells significantly, it also exhibited cytotoxicity towards normal cells, highlighting the need for further optimization to minimize side effects .
Q & A
Q. Basic: What synthetic methodologies are reliable for producing 3-Acetyl-4,5-dimethyl-1H-pyrrole-2-carboxylic acid?
Answer:
The compound is commonly synthesized via oxidation of its ethyl ester derivative (e.g., Ethyl 4-acetyl-3,5-dimethyl-1H-pyrrole-2-carboxylate) using potassium permanganate (KMnO₄) under acidic or neutral conditions. Post-reaction, the product is purified via recrystallization or column chromatography. Alternative routes include hydrolysis of acetyl-protected precursors under controlled pH conditions. Comparative studies show that the ethyl ester derivative serves as a stable intermediate for subsequent functionalization .
Table 1: Key Synthetic Routes
Method | Reagents/Conditions | Key Intermediate |
---|---|---|
Oxidation | KMnO₄ (acidic/neutral) | Ethyl ester derivative |
Hydrolysis | NaOH/HCl, aqueous conditions | Acetyl-protected precursors |
Q. Basic: How is the structural integrity of this compound validated in research settings?
Answer:
Structural confirmation relies on spectroscopic and crystallographic techniques :
- NMR : Distinct signals for acetyl (δ ~2.5 ppm, singlet) and carboxylic acid (δ ~12 ppm, broad) groups.
- X-ray crystallography : Resolves hydrogen-bonding networks (e.g., centrosymmetric dimers via N–H⋯O interactions, as seen in related pyrrole-carboxylic acids) .
- IR spectroscopy : Stretching vibrations for C=O (~1700 cm⁻¹) and O–H (~2500-3000 cm⁻¹) confirm functional groups.
Q. Advanced: How do structural modifications at the acetyl or methyl groups influence bioactivity?
Answer:
Modifications alter electronic and steric properties, impacting interactions with biological targets:
- Acetyl group removal (e.g., Ethyl 3,5-dimethyl-1H-pyrrole-2-carboxylate) reduces electrophilicity, diminishing reactivity in nucleophilic assays.
- Formyl substitution (e.g., Ethyl 4-formyl-3,5-dimethyl-1H-pyrrole-2-carboxylate) increases polarity but may destabilize the pyrrole ring.
- Methyl group position : 3,5-dimethyl substitution enhances steric hindrance, affecting binding to enzyme active sites (e.g., kinase inhibitors) .
Table 2: Bioactivity of Structural Analogs
Compound | Key Modification | Observed Bioactivity |
---|---|---|
4-Acetyl-3,5-dimethyl-1H-pyrrole-2-carboxylic acid | Native structure | Moderate kinase inhibition |
Ethyl 3,5-dimethyl-1H-pyrrole-2-carboxylate | Acetyl group removed | Reduced cytotoxicity |
Ethyl 4-formyl-3,5-dimethyl-1H-pyrrole-2-carboxylate | Acetyl → formyl | Enhanced solubility, lower stability |
Q. Advanced: What strategies resolve contradictions in reported biological activity data?
Answer:
Discrepancies often arise from assay variability or impurity profiles . Mitigation strategies include:
- Purity validation : HPLC (>95% purity) and mass spectrometry to exclude byproducts.
- Standardized assays : Use consistent cell lines (e.g., HEK293 for kinase assays) and controls.
- Comparative studies : Benchmark against structurally characterized analogs (e.g., free acid vs. ester derivatives) to isolate substituent effects .
Q. Advanced: How can computational modeling predict this compound’s interaction with protein targets?
Answer:
Molecular docking (e.g., AutoDock Vina) and QSAR models are employed to:
- Identify binding poses in enzymatic pockets (e.g., ATP-binding sites of kinases).
- Predict pharmacokinetic properties (e.g., logP for membrane permeability).
- Validate with MD simulations to assess binding stability over time. For example, the acetyl group’s orientation may influence hydrogen bonding with conserved lysine residues in kinases .
Q. Advanced: What experimental designs optimize solubility for in vivo studies?
Answer:
- Salt formation : React with sodium bicarbonate to produce water-soluble carboxylate salts.
- Co-solvents : Use DMSO:water mixtures (<10% DMSO) to maintain compound stability.
- Prodrug strategies : Synthesize ester derivatives (e.g., ethyl ester) for improved lipophilicity, followed by enzymatic hydrolysis in vivo .
Q. Basic: What are the key challenges in scaling up synthesis without compromising yield?
Answer:
Properties
IUPAC Name |
3-acetyl-4,5-dimethyl-1H-pyrrole-2-carboxylic acid | |
---|---|---|
Source | PubChem | |
URL | https://pubchem.ncbi.nlm.nih.gov | |
Description | Data deposited in or computed by PubChem | |
InChI |
InChI=1S/C9H11NO3/c1-4-5(2)10-8(9(12)13)7(4)6(3)11/h10H,1-3H3,(H,12,13) | |
Source | PubChem | |
URL | https://pubchem.ncbi.nlm.nih.gov | |
Description | Data deposited in or computed by PubChem | |
InChI Key |
PPSGKMWFWAXDHT-UHFFFAOYSA-N | |
Source | PubChem | |
URL | https://pubchem.ncbi.nlm.nih.gov | |
Description | Data deposited in or computed by PubChem | |
Canonical SMILES |
CC1=C(NC(=C1C(=O)C)C(=O)O)C | |
Source | PubChem | |
URL | https://pubchem.ncbi.nlm.nih.gov | |
Description | Data deposited in or computed by PubChem | |
Molecular Formula |
C9H11NO3 | |
Source | PubChem | |
URL | https://pubchem.ncbi.nlm.nih.gov | |
Description | Data deposited in or computed by PubChem | |
DSSTOX Substance ID |
DTXSID80343583 | |
Record name | 3-Acetyl-4,5-dimethyl-1H-pyrrole-2-carboxylic acid | |
Source | EPA DSSTox | |
URL | https://comptox.epa.gov/dashboard/DTXSID80343583 | |
Description | DSSTox provides a high quality public chemistry resource for supporting improved predictive toxicology. | |
Molecular Weight |
181.19 g/mol | |
Source | PubChem | |
URL | https://pubchem.ncbi.nlm.nih.gov | |
Description | Data deposited in or computed by PubChem | |
CAS No. |
2386-33-6 | |
Record name | 3-Acetyl-4,5-dimethyl-1H-pyrrole-2-carboxylic acid | |
Source | CAS Common Chemistry | |
URL | https://commonchemistry.cas.org/detail?cas_rn=2386-33-6 | |
Description | CAS Common Chemistry is an open community resource for accessing chemical information. Nearly 500,000 chemical substances from CAS REGISTRY cover areas of community interest, including common and frequently regulated chemicals, and those relevant to high school and undergraduate chemistry classes. This chemical information, curated by our expert scientists, is provided in alignment with our mission as a division of the American Chemical Society. | |
Explanation | The data from CAS Common Chemistry is provided under a CC-BY-NC 4.0 license, unless otherwise stated. | |
Record name | 3-Acetyl-4,5-dimethyl-1H-pyrrole-2-carboxylic acid | |
Source | EPA DSSTox | |
URL | https://comptox.epa.gov/dashboard/DTXSID80343583 | |
Description | DSSTox provides a high quality public chemistry resource for supporting improved predictive toxicology. | |
Synthesis routes and methods
Procedure details
Retrosynthesis Analysis
AI-Powered Synthesis Planning: Our tool employs the Template_relevance Pistachio, Template_relevance Bkms_metabolic, Template_relevance Pistachio_ringbreaker, Template_relevance Reaxys, Template_relevance Reaxys_biocatalysis model, leveraging a vast database of chemical reactions to predict feasible synthetic routes.
One-Step Synthesis Focus: Specifically designed for one-step synthesis, it provides concise and direct routes for your target compounds, streamlining the synthesis process.
Accurate Predictions: Utilizing the extensive PISTACHIO, BKMS_METABOLIC, PISTACHIO_RINGBREAKER, REAXYS, REAXYS_BIOCATALYSIS database, our tool offers high-accuracy predictions, reflecting the latest in chemical research and data.
Strategy Settings
Precursor scoring | Relevance Heuristic |
---|---|
Min. plausibility | 0.01 |
Model | Template_relevance |
Template Set | Pistachio/Bkms_metabolic/Pistachio_ringbreaker/Reaxys/Reaxys_biocatalysis |
Top-N result to add to graph | 6 |
Feasible Synthetic Routes
Disclaimer and Information on In-Vitro Research Products
Please be aware that all articles and product information presented on BenchChem are intended solely for informational purposes. The products available for purchase on BenchChem are specifically designed for in-vitro studies, which are conducted outside of living organisms. In-vitro studies, derived from the Latin term "in glass," involve experiments performed in controlled laboratory settings using cells or tissues. It is important to note that these products are not categorized as medicines or drugs, and they have not received approval from the FDA for the prevention, treatment, or cure of any medical condition, ailment, or disease. We must emphasize that any form of bodily introduction of these products into humans or animals is strictly prohibited by law. It is essential to adhere to these guidelines to ensure compliance with legal and ethical standards in research and experimentation.