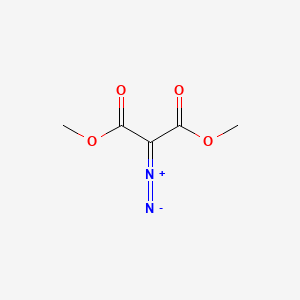
Dimethyl diazomalonate
Overview
Description
Dimethyl diazomalonate is an organic compound characterized by the presence of two ester groups and a diazo group. It is a versatile reagent in organic synthesis, particularly valued for its ability to generate carbenes, which are highly reactive intermediates used in various chemical transformations.
Mechanism of Action
Target of Action
Dimethyl diazomalonate is a diazo compound that primarily targets carbon atoms in organic molecules . The compound’s primary targets are the carbon atoms in alcohols and in non-activated double bonds of fatty esters . These carbon atoms play a crucial role in the structure and function of these organic molecules.
Mode of Action
This compound interacts with its targets through a process known as carbene insertion . In this process, the diazo group in this compound decomposes to generate a carbene, which is a highly reactive species consisting of a carbon atom with two non-bonded electrons . This carbene then inserts itself into a carbon-hydrogen bond in the target molecule . The result is the formation of new carbon-carbon bonds, leading to structural changes in the target molecule .
Biochemical Pathways
The carbene insertion process affects various biochemical pathways. For instance, the insertion of biscarbomethoxycarbene into alcohols leads to the formation of O-H insertion products . Similarly, the reaction of this compound with non-activated double bonds of fatty esters results in the formation of cyclopropanes . These new compounds can further participate in various biochemical reactions, leading to downstream effects on multiple biochemical pathways .
Result of Action
The action of this compound at the molecular level results in the formation of new compounds with altered structures . These structural changes can affect the properties and functions of the original molecules. For example, the formation of O-H insertion products from alcohols can change the alcohols’ reactivity . Similarly, the formation of cyclopropanes from fatty esters can alter the esters’ physical and chemical properties .
Action Environment
The action of this compound is influenced by various environmental factors. For instance, the presence of a catalyst such as rhodium or copper can enhance the compound’s reactivity . The temperature also affects the compound’s reactivity, with higher temperatures promoting the thermal rearrangement of the products formed from this compound . Moreover, the compound’s stability and efficacy can be affected by factors such as pH, the presence of other chemicals, and the specific conditions of the reaction environment .
Biochemical Analysis
Biochemical Properties
Dimethyl diazomalonate plays a significant role in biochemical reactions. It interacts with enzymes, proteins, and other biomolecules, particularly those with carboxylic acid groups . The nature of these interactions is largely dependent on the basicity of the diazo compound .
Molecular Mechanism
The mechanism of action of this compound involves the conversion of carboxylic acids to esters . This process occurs efficiently in an aqueous environment and extends to carboxylic acid groups in a protein . The ensuing esters are hydrolyzed by human cellular esterases to regenerate protein carboxyl groups .
Preparation Methods
Synthetic Routes and Reaction Conditions: Dimethyl diazomalonate can be synthesized through the reaction of dimethyl malonate with 4-acetamidobenzenesulfonyl azide in the presence of triethylamine and acetonitrile. The reaction mixture is cooled to 0°C, and dimethyl malonate is added slowly. The mixture is then stirred at room temperature for 17 hours, resulting in the formation of this compound as a yellow oil .
Industrial Production Methods: The industrial production of this compound follows similar synthetic routes but on a larger scale. The process involves careful control of reaction conditions to ensure safety, given the explosive nature of diazo compounds. The crude product is typically purified by distillation under reduced pressure .
Chemical Reactions Analysis
Types of Reactions: Dimethyl diazomalonate undergoes various types of reactions, including:
N-H Insertion Reactions: It reacts with aliphatic and aromatic amines in the presence of iridium catalysts to form N-substituted products.
Cycloaddition Reactions: It participates in 1,3-dipolar cycloaddition reactions with alkenes and alkynes to form five-membered heterocycles.
Common Reagents and Conditions:
Catalysts: Rhodium (II) acetate, iridium complexes, and other transition metal catalysts are commonly used.
Solvents: Acetonitrile, chloroform, and dichloromethane are frequently employed.
Reaction Conditions: Reactions are typically carried out at room temperature or under mild heating
Major Products:
N-H Insertion Products: Formation of N-substituted amines.
Cycloaddition Products: Formation of heterocyclic compounds such as oxazoles
Scientific Research Applications
Dimethyl diazomalonate is widely used in scientific research due to its versatility:
Chemistry: It is used in the synthesis of complex organic molecules, including heterocycles and natural products.
Biology: It is employed in the functionalization of biomolecules and the study of enzyme mechanisms.
Medicine: It serves as a precursor in the synthesis of pharmaceutical compounds.
Industry: It is used in the production of fine chemicals and materials with specific properties.
Comparison with Similar Compounds
Ethyl diazoacetate: Another diazo compound used for carbene generation.
Diazomethane: A simpler diazo compound with similar reactivity but higher explosiveness.
Comparison:
Reactivity: Dimethyl diazomalonate is more stable than diazomethane and can be handled more safely.
Applications: While both this compound and ethyl diazoacetate are used in carbene chemistry, this compound offers greater versatility in forming N-H insertion products and heterocycles
This compound stands out due to its stability, versatility, and wide range of applications in scientific research and industrial processes.
Properties
IUPAC Name |
dimethyl 2-diazopropanedioate | |
---|---|---|
Source | PubChem | |
URL | https://pubchem.ncbi.nlm.nih.gov | |
Description | Data deposited in or computed by PubChem | |
InChI |
InChI=1S/C5H6N2O4/c1-10-4(8)3(7-6)5(9)11-2/h1-2H3 | |
Source | PubChem | |
URL | https://pubchem.ncbi.nlm.nih.gov | |
Description | Data deposited in or computed by PubChem | |
InChI Key |
UFAZHCVBGMSJHS-UHFFFAOYSA-N | |
Source | PubChem | |
URL | https://pubchem.ncbi.nlm.nih.gov | |
Description | Data deposited in or computed by PubChem | |
Canonical SMILES |
COC(=O)C(=[N+]=[N-])C(=O)OC | |
Source | PubChem | |
URL | https://pubchem.ncbi.nlm.nih.gov | |
Description | Data deposited in or computed by PubChem | |
Molecular Formula |
C5H6N2O4 | |
Source | PubChem | |
URL | https://pubchem.ncbi.nlm.nih.gov | |
Description | Data deposited in or computed by PubChem | |
DSSTOX Substance ID |
DTXSID40987068 | |
Record name | 2-Diazonio-1,3-dimethoxy-3-oxoprop-1-en-1-olate | |
Source | EPA DSSTox | |
URL | https://comptox.epa.gov/dashboard/DTXSID40987068 | |
Description | DSSTox provides a high quality public chemistry resource for supporting improved predictive toxicology. | |
Molecular Weight |
158.11 g/mol | |
Source | PubChem | |
URL | https://pubchem.ncbi.nlm.nih.gov | |
Description | Data deposited in or computed by PubChem | |
CAS No. |
6773-29-1 | |
Record name | Dimethyl diazomalonate | |
Source | DTP/NCI | |
URL | https://dtp.cancer.gov/dtpstandard/servlet/dwindex?searchtype=NSC&outputformat=html&searchlist=138651 | |
Description | The NCI Development Therapeutics Program (DTP) provides services and resources to the academic and private-sector research communities worldwide to facilitate the discovery and development of new cancer therapeutic agents. | |
Explanation | Unless otherwise indicated, all text within NCI products is free of copyright and may be reused without our permission. Credit the National Cancer Institute as the source. | |
Record name | 2-Diazonio-1,3-dimethoxy-3-oxoprop-1-en-1-olate | |
Source | EPA DSSTox | |
URL | https://comptox.epa.gov/dashboard/DTXSID40987068 | |
Description | DSSTox provides a high quality public chemistry resource for supporting improved predictive toxicology. | |
Synthesis routes and methods
Procedure details
Retrosynthesis Analysis
AI-Powered Synthesis Planning: Our tool employs the Template_relevance Pistachio, Template_relevance Bkms_metabolic, Template_relevance Pistachio_ringbreaker, Template_relevance Reaxys, Template_relevance Reaxys_biocatalysis model, leveraging a vast database of chemical reactions to predict feasible synthetic routes.
One-Step Synthesis Focus: Specifically designed for one-step synthesis, it provides concise and direct routes for your target compounds, streamlining the synthesis process.
Accurate Predictions: Utilizing the extensive PISTACHIO, BKMS_METABOLIC, PISTACHIO_RINGBREAKER, REAXYS, REAXYS_BIOCATALYSIS database, our tool offers high-accuracy predictions, reflecting the latest in chemical research and data.
Strategy Settings
Precursor scoring | Relevance Heuristic |
---|---|
Min. plausibility | 0.01 |
Model | Template_relevance |
Template Set | Pistachio/Bkms_metabolic/Pistachio_ringbreaker/Reaxys/Reaxys_biocatalysis |
Top-N result to add to graph | 6 |
Feasible Synthetic Routes
Q1: What is the molecular formula and weight of dimethyl diazomalonate?
A1: The molecular formula of this compound is C5H6N2O4 and its molecular weight is 158.11 g/mol.
Q2: Are there any spectroscopic data available for this compound?
A2: Yes, infrared (IR) spectroscopy reveals a characteristic strong absorption band for the diazo group (N2) around 1911 cm-1. []
Q3: What types of reactions is this compound commonly used for?
A3: this compound is primarily used as a carbene precursor in reactions catalyzed by transition metals, particularly copper and rhodium complexes. Common reactions include cyclopropanation of alkenes, insertion into various bonds (C-H, N-H, Se-Se), and formation of ylides.
Q4: How do catalysts influence the reactions of this compound?
A5: Transition metal catalysts like rhodium(II) acetate [] or copper(II) acetylacetonate [] decompose this compound to generate reactive metal-carbenoid intermediates. These intermediates can then participate in various reactions depending on the reaction conditions and the other reactants present.
Q5: Can you provide an example of a cyclopropanation reaction using this compound?
A6: this compound readily undergoes cyclopropanation with alkenes in the presence of a rhodium(II) catalyst, like [Rh2(esp)2], even with low catalyst loading and the alkene as the limiting reagent. []
Q6: What about reactions with thioketones?
A7: this compound reacts with aromatic thioketones in the presence of Rh2(OAc)4, producing a mixture of thiiranedicarboxylate and (diphenylmethylidene)malonate. The proposed mechanism involves a thiocarbonylylide intermediate. []
Q7: What are some other interesting reactions involving this compound?
A8:
Insertion into Se-Se bonds: In the presence of copper-bronze or BF3·Et2O, this compound inserts into the Se-Se bond of diphenyl diselenide. []* Formation of thiophenium ylides: Rhodium(II) acetate catalyzes the addition of this compound to thiophene and its derivatives, yielding stable thiophenium ylides. []* Synthesis of oxazoles: BF3 catalyzes the reaction of this compound with nitriles to synthesize oxazoles. This reaction proceeds via betaine intermediates. []* Formation of sulfonium ylides:* this compound reacts with dialkyl sulfides in the presence of catalytic amounts of dialkoxy disulfides to form sulfonium ylides. []
Q8: Has computational chemistry been used to study this compound reactions?
A9: Yes, density functional theory (DFT) calculations have been employed to study the mechanism of copper(I)-catalyzed cyclopropanation reactions of this compound with alkenes. These studies provide insights into the energetics and intermediates involved in the reaction pathway. []
Disclaimer and Information on In-Vitro Research Products
Please be aware that all articles and product information presented on BenchChem are intended solely for informational purposes. The products available for purchase on BenchChem are specifically designed for in-vitro studies, which are conducted outside of living organisms. In-vitro studies, derived from the Latin term "in glass," involve experiments performed in controlled laboratory settings using cells or tissues. It is important to note that these products are not categorized as medicines or drugs, and they have not received approval from the FDA for the prevention, treatment, or cure of any medical condition, ailment, or disease. We must emphasize that any form of bodily introduction of these products into humans or animals is strictly prohibited by law. It is essential to adhere to these guidelines to ensure compliance with legal and ethical standards in research and experimentation.