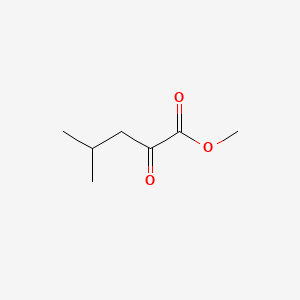
Methyl 4-methyl-2-oxopentanoate
Overview
Description
Preparation Methods
Synthetic Routes and Reaction Conditions
Methyl 4-methyl-2-oxopentanoate can be synthesized through several methods. One common approach involves the esterification of 4-methyl-2-oxopentanoic acid with methanol in the presence of an acid catalyst such as sulfuric acid. The reaction is typically carried out under reflux conditions to ensure complete conversion of the acid to its ester form.
Industrial Production Methods
In an industrial setting, the production of this compound may involve more efficient and scalable processes. These could include continuous flow reactors and the use of alternative catalysts to optimize yield and reduce production costs. The choice of method depends on factors such as the desired purity, production scale, and economic considerations.
Chemical Reactions Analysis
Types of Reactions
Methyl 4-methyl-2-oxopentanoate undergoes various chemical reactions, including:
Oxidation: The compound can be oxidized to form corresponding carboxylic acids or other oxidized derivatives.
Reduction: Reduction reactions can convert the keto group to a hydroxyl group, yielding alcohol derivatives.
Substitution: The ester group can participate in nucleophilic substitution reactions, leading to the formation of different ester or amide derivatives.
Common Reagents and Conditions
Oxidation: Common oxidizing agents include potassium permanganate (KMnO4) and chromium trioxide (CrO3).
Reduction: Reducing agents such as sodium borohydride (NaBH4) or lithium aluminum hydride (LiAlH4) are often used.
Substitution: Nucleophiles like amines or alcohols can react with the ester group under acidic or basic conditions.
Major Products Formed
The major products formed from these reactions depend on the specific reagents and conditions used. For example, oxidation may yield 4-methyl-2-oxopentanoic acid, while reduction could produce 4-methyl-2-hydroxypentanoate.
Scientific Research Applications
Methyl 4-methyl-2-oxopentanoate has a wide range of applications in scientific research:
Chemistry: It is used as a building block in organic synthesis, particularly in the preparation of more complex molecules.
Biology: The compound is studied for its role in metabolic pathways, especially in the context of amino acid metabolism.
Medicine: Research explores its potential as a precursor for pharmaceuticals and its effects on biological systems.
Industry: It is utilized in the production of various chemicals and materials, including solvents and intermediates for polymer synthesis.
Mechanism of Action
The mechanism by which methyl 4-methyl-2-oxopentanoate exerts its effects involves its interaction with specific molecular targets and pathways. For instance, in biological systems, it may participate in enzymatic reactions that convert it to other metabolites. These processes often involve the transfer of functional groups and changes in redox states, influencing cellular metabolism and signaling pathways.
Comparison with Similar Compounds
Similar Compounds
4-Methyl-2-oxopentanoic acid: The parent acid form of the ester.
α-Ketoisocaproic acid: Another name for 4-methyl-2-oxopentanoic acid.
2-Oxoisocaproate: A synonym for the same compound.
Uniqueness
Methyl 4-methyl-2-oxopentanoate is unique due to its ester functional group, which imparts different chemical reactivity and physical properties compared to its acid counterpart. This makes it valuable in specific synthetic applications where the ester group can be selectively modified.
Properties
IUPAC Name |
methyl 4-methyl-2-oxopentanoate | |
---|---|---|
Source | PubChem | |
URL | https://pubchem.ncbi.nlm.nih.gov | |
Description | Data deposited in or computed by PubChem | |
InChI |
InChI=1S/C7H12O3/c1-5(2)4-6(8)7(9)10-3/h5H,4H2,1-3H3 | |
Source | PubChem | |
URL | https://pubchem.ncbi.nlm.nih.gov | |
Description | Data deposited in or computed by PubChem | |
InChI Key |
LFVDEVQENPHSSD-UHFFFAOYSA-N | |
Source | PubChem | |
URL | https://pubchem.ncbi.nlm.nih.gov | |
Description | Data deposited in or computed by PubChem | |
Canonical SMILES |
CC(C)CC(=O)C(=O)OC | |
Source | PubChem | |
URL | https://pubchem.ncbi.nlm.nih.gov | |
Description | Data deposited in or computed by PubChem | |
Molecular Formula |
C7H12O3 | |
Source | PubChem | |
URL | https://pubchem.ncbi.nlm.nih.gov | |
Description | Data deposited in or computed by PubChem | |
DSSTOX Substance ID |
DTXSID60338058 | |
Record name | Methyl 4-methyl-2-oxopentanoate | |
Source | EPA DSSTox | |
URL | https://comptox.epa.gov/dashboard/DTXSID60338058 | |
Description | DSSTox provides a high quality public chemistry resource for supporting improved predictive toxicology. | |
Molecular Weight |
144.17 g/mol | |
Source | PubChem | |
URL | https://pubchem.ncbi.nlm.nih.gov | |
Description | Data deposited in or computed by PubChem | |
CAS No. |
3682-43-7 | |
Record name | Methyl 4-methyl-2-oxopentanoate | |
Source | EPA DSSTox | |
URL | https://comptox.epa.gov/dashboard/DTXSID60338058 | |
Description | DSSTox provides a high quality public chemistry resource for supporting improved predictive toxicology. | |
Synthesis routes and methods
Procedure details
Retrosynthesis Analysis
AI-Powered Synthesis Planning: Our tool employs the Template_relevance Pistachio, Template_relevance Bkms_metabolic, Template_relevance Pistachio_ringbreaker, Template_relevance Reaxys, Template_relevance Reaxys_biocatalysis model, leveraging a vast database of chemical reactions to predict feasible synthetic routes.
One-Step Synthesis Focus: Specifically designed for one-step synthesis, it provides concise and direct routes for your target compounds, streamlining the synthesis process.
Accurate Predictions: Utilizing the extensive PISTACHIO, BKMS_METABOLIC, PISTACHIO_RINGBREAKER, REAXYS, REAXYS_BIOCATALYSIS database, our tool offers high-accuracy predictions, reflecting the latest in chemical research and data.
Strategy Settings
Precursor scoring | Relevance Heuristic |
---|---|
Min. plausibility | 0.01 |
Model | Template_relevance |
Template Set | Pistachio/Bkms_metabolic/Pistachio_ringbreaker/Reaxys/Reaxys_biocatalysis |
Top-N result to add to graph | 6 |
Feasible Synthetic Routes
Disclaimer and Information on In-Vitro Research Products
Please be aware that all articles and product information presented on BenchChem are intended solely for informational purposes. The products available for purchase on BenchChem are specifically designed for in-vitro studies, which are conducted outside of living organisms. In-vitro studies, derived from the Latin term "in glass," involve experiments performed in controlled laboratory settings using cells or tissues. It is important to note that these products are not categorized as medicines or drugs, and they have not received approval from the FDA for the prevention, treatment, or cure of any medical condition, ailment, or disease. We must emphasize that any form of bodily introduction of these products into humans or animals is strictly prohibited by law. It is essential to adhere to these guidelines to ensure compliance with legal and ethical standards in research and experimentation.