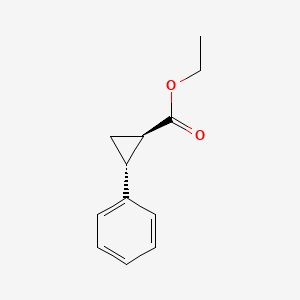
Ethyl trans-2-phenylcyclopropanecarboxylate
Overview
Description
Ethyl trans-2-phenylcyclopropanecarboxylate is an organic compound with the molecular formula C12H14O2. It is a cyclopropane derivative, characterized by a three-membered ring structure with a phenyl group and an ethyl ester group attached.
Preparation Methods
Synthetic Routes and Reaction Conditions: Ethyl trans-2-phenylcyclopropanecarboxylate can be synthesized through the reaction of ethyl diazoacetate with styrene in the presence of a catalyst. The reaction is typically carried out in a solvent such as xylene, under reflux conditions. The mixture is then distilled to obtain the desired product .
Industrial Production Methods: In industrial settings, the synthesis of this compound follows similar principles but on a larger scale. The use of continuous flow reactors and optimized reaction conditions can enhance yield and efficiency. The product is purified through distillation and crystallization techniques .
Chemical Reactions Analysis
Types of Reactions: Ethyl trans-2-phenylcyclopropanecarboxylate undergoes various chemical reactions, including:
Oxidation: The compound can be oxidized to form corresponding carboxylic acids.
Reduction: Reduction reactions can convert the ester group to an alcohol.
Substitution: The phenyl group can participate in electrophilic aromatic substitution reactions.
Common Reagents and Conditions:
Oxidation: Common oxidizing agents include potassium permanganate (KMnO4) and chromium trioxide (CrO3).
Reduction: Reducing agents such as lithium aluminum hydride (LiAlH4) are used.
Substitution: Reagents like bromine (Br2) and nitric acid (HNO3) are employed for substitution reactions.
Major Products Formed:
Oxidation: Phenylcyclopropanecarboxylic acid.
Reduction: Ethyl trans-2-phenylcyclopropanol.
Substitution: Various substituted phenyl derivatives.
Scientific Research Applications
Ethyl trans-2-phenylcyclopropanecarboxylate has diverse applications in scientific research:
Chemistry: It is used as a building block in organic synthesis and as a precursor for more complex molecules.
Biology: The compound is studied for its potential biological activities, including antimicrobial properties.
Medicine: Research is ongoing to explore its potential as a pharmaceutical intermediate.
Industry: It is used in the synthesis of specialty chemicals and materials.
Mechanism of Action
The mechanism of action of ethyl trans-2-phenylcyclopropanecarboxylate involves its interaction with various molecular targets. The cyclopropane ring’s strained nature makes it highly reactive, allowing it to participate in ring-opening reactions and other transformations. These reactions can modulate biological pathways and affect cellular functions .
Comparison with Similar Compounds
- Ethyl cis-2-phenylcyclopropanecarboxylate
- Cyclopropanecarboxylic acid, 2-phenyl-, ethyl ester
- Ethyl 2-phenylcyclopropanecarboxylate
Comparison: Ethyl trans-2-phenylcyclopropanecarboxylate is unique due to its trans configuration, which imparts distinct chemical and physical properties compared to its cis isomer. The trans isomer typically exhibits different reactivity and stability, making it suitable for specific applications .
Properties
IUPAC Name |
ethyl (1R,2R)-2-phenylcyclopropane-1-carboxylate | |
---|---|---|
Source | PubChem | |
URL | https://pubchem.ncbi.nlm.nih.gov | |
Description | Data deposited in or computed by PubChem | |
InChI |
InChI=1S/C12H14O2/c1-2-14-12(13)11-8-10(11)9-6-4-3-5-7-9/h3-7,10-11H,2,8H2,1H3/t10-,11+/m0/s1 | |
Source | PubChem | |
URL | https://pubchem.ncbi.nlm.nih.gov | |
Description | Data deposited in or computed by PubChem | |
InChI Key |
SRGUIJLJERBBCM-WDEREUQCSA-N | |
Source | PubChem | |
URL | https://pubchem.ncbi.nlm.nih.gov | |
Description | Data deposited in or computed by PubChem | |
Canonical SMILES |
CCOC(=O)C1CC1C2=CC=CC=C2 | |
Source | PubChem | |
URL | https://pubchem.ncbi.nlm.nih.gov | |
Description | Data deposited in or computed by PubChem | |
Isomeric SMILES |
CCOC(=O)[C@@H]1C[C@H]1C2=CC=CC=C2 | |
Source | PubChem | |
URL | https://pubchem.ncbi.nlm.nih.gov | |
Description | Data deposited in or computed by PubChem | |
Molecular Formula |
C12H14O2 | |
Source | PubChem | |
URL | https://pubchem.ncbi.nlm.nih.gov | |
Description | Data deposited in or computed by PubChem | |
Molecular Weight |
190.24 g/mol | |
Source | PubChem | |
URL | https://pubchem.ncbi.nlm.nih.gov | |
Description | Data deposited in or computed by PubChem | |
CAS No. |
946-39-4 | |
Record name | Ethyl trans-2-phenylcyclopropanecarboxylate | |
Source | DTP/NCI | |
URL | https://dtp.cancer.gov/dtpstandard/servlet/dwindex?searchtype=NSC&outputformat=html&searchlist=74491 | |
Description | The NCI Development Therapeutics Program (DTP) provides services and resources to the academic and private-sector research communities worldwide to facilitate the discovery and development of new cancer therapeutic agents. | |
Explanation | Unless otherwise indicated, all text within NCI products is free of copyright and may be reused without our permission. Credit the National Cancer Institute as the source. | |
Synthesis routes and methods I
Procedure details
Synthesis routes and methods II
Procedure details
Synthesis routes and methods III
Procedure details
Synthesis routes and methods IV
Procedure details
Retrosynthesis Analysis
AI-Powered Synthesis Planning: Our tool employs the Template_relevance Pistachio, Template_relevance Bkms_metabolic, Template_relevance Pistachio_ringbreaker, Template_relevance Reaxys, Template_relevance Reaxys_biocatalysis model, leveraging a vast database of chemical reactions to predict feasible synthetic routes.
One-Step Synthesis Focus: Specifically designed for one-step synthesis, it provides concise and direct routes for your target compounds, streamlining the synthesis process.
Accurate Predictions: Utilizing the extensive PISTACHIO, BKMS_METABOLIC, PISTACHIO_RINGBREAKER, REAXYS, REAXYS_BIOCATALYSIS database, our tool offers high-accuracy predictions, reflecting the latest in chemical research and data.
Strategy Settings
Precursor scoring | Relevance Heuristic |
---|---|
Min. plausibility | 0.01 |
Model | Template_relevance |
Template Set | Pistachio/Bkms_metabolic/Pistachio_ringbreaker/Reaxys/Reaxys_biocatalysis |
Top-N result to add to graph | 6 |
Feasible Synthetic Routes
Q1: What is the significance of Ethyl trans-2-phenylcyclopropanecarboxylate in the context of the research?
A1: this compound serves as a valuable product in a standard test reaction for homogeneous catalysts that facilitate cyclopropanation. [] Researchers utilize the reaction between styrene and ethyl diazoacetate (EDA), examining the catalytic activity of various systems in producing this specific diastereomer. This helps assess the catalyst's selectivity towards the trans isomer. []
Disclaimer and Information on In-Vitro Research Products
Please be aware that all articles and product information presented on BenchChem are intended solely for informational purposes. The products available for purchase on BenchChem are specifically designed for in-vitro studies, which are conducted outside of living organisms. In-vitro studies, derived from the Latin term "in glass," involve experiments performed in controlled laboratory settings using cells or tissues. It is important to note that these products are not categorized as medicines or drugs, and they have not received approval from the FDA for the prevention, treatment, or cure of any medical condition, ailment, or disease. We must emphasize that any form of bodily introduction of these products into humans or animals is strictly prohibited by law. It is essential to adhere to these guidelines to ensure compliance with legal and ethical standards in research and experimentation.