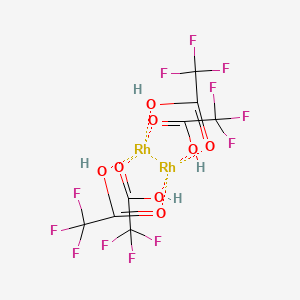
Rhodium(II) trifluoroacetate dimer
Overview
Description
Rhodium(II) trifluoroacetate dimer (chemical formula: C₈F₁₂O₈Rh₂, CAS 31126-95-1) is a dirhodium complex where two rhodium atoms are bridged by four trifluoroacetate ligands. It is a blue-green crystalline powder with a molecular weight of 657.87 g/mol and rhodium content ranging from 27.5% to 35.0% .
Preparation Methods
Rhodium(II) trifluoroacetate dimer is typically synthesized by dissolving rhodium(II) acetate in hot trifluoroacetic acid. The reaction can be represented as follows :
[ \text{Rh}_2(\text{O}_2\text{CCH}_3)_4 + 4 \text{HO}_2\text{CCF}_3 \rightarrow \text{Rh}_2(\text{O}_2\text{CCF}_3)_4 + 4 \text{HO}_2\text{CCH}_3 ]
This reaction expels acetic acid and retains the Rh-Rh bond. The compound can also form adducts with various Lewis bases, often with a 2:1 stoichiometry .
Chemical Reactions Analysis
Rhodium(II) trifluoroacetate dimer undergoes several types of chemical reactions, including:
Cyclopropanation of Alkenes: This reaction is catalyzed by rhodium(II) trifluoroacetate using diazo compounds.
[ \text{RCH=CR’H} + \text{CH}_3\text{CH}_2\text{O}_2\text{CCH(N}_2) \rightarrow \text{cyclo-(RCH)(R’CH)(CH}_3\text{CH}_2\text{O}_2\text{CCH)} + \text{N}_2 ]
Scientific Research Applications
Chemical Properties and Structure
- Molecular Formula : C₈F₁₂O₈Rh₂
- Molecular Weight : 657.87 g/mol
- IUPAC Name : Rhodium(2+); 2,2,2-trifluoroacetate
- Structure : The compound exhibits a unique "Chinese lantern" structure characterized by a Rh-Rh bond, contributing to its diamagnetic properties and reactivity with Lewis bases.
Catalytic Applications
Rhodium(II) trifluoroacetate dimer serves as a catalyst in various organic transformations:
- Cyclopropanation Reactions : It effectively catalyzes cyclopropanation of alkenes using diazo compounds, which is crucial for synthesizing complex organic molecules.
- Synthesis of α,β-Unsaturated Carbonyl Compounds : The compound is utilized to produce isomerically pure α,β-unsaturated carbonyl compounds, which are important intermediates in organic synthesis .
- Formation of Adducts with Lewis Bases : The dimer interacts with Lewis bases at the axial positions of the rhodium centers, enhancing electrophilicity and facilitating various reactions.
Industrial Applications
The compound's catalytic properties extend to industrial applications:
- Pharmaceutical Development : this compound is leveraged in the synthesis of biologically active molecules and pharmaceuticals, contributing to advancements in medicinal chemistry .
- Materials Science : Its role in producing organic compounds aids in the development of new materials and industrial processes.
Case Study 1: Cyclopropanation Efficiency
A study demonstrated that Rh₂(O₂CCF₃)₄ outperformed other catalysts in cyclopropanation reactions involving ethyl diazoacetate. The reaction conditions were optimized to yield high selectivity and efficiency, underscoring the compound's potential in synthetic organic chemistry .
Case Study 2: Pharmaceutical Synthesis
In pharmaceutical research, Rh₂(O₂CCF₃)₄ has been utilized to synthesize complex molecules that exhibit biological activity. The ability to form stable adducts with various substrates has facilitated the development of novel therapeutic agents .
Mechanism of Action
The mechanism of action of rhodium(II) trifluoroacetate dimer involves its ability to form adducts with Lewis bases and catalyze cyclopropanation reactions. The Rh-Rh bond plays a crucial role in these processes, facilitating the formation of reactive intermediates that drive the chemical reactions .
Comparison with Similar Compounds
Comparison with Similar Rhodium(II) Carboxylates
Rhodium(II) Acetate Dimer (Rh₂(OAc)₄)
Rhodium(II) Citrate (Rh₂Cit)
Trinuclear Rhodium Acetate (Rh₃(OAc)₆)
Research Findings and Performance Data
Catalytic Hydrogenation
- Rh₂(COOCF₃)₄ outperforms Rh₂(OAc)₄ in hydrogenating nitroarenes (e.g., o-nitrophenol) due to enhanced electron withdrawal by CF₃ groups, achieving >95% conversion under mild conditions .
- Rh₂Cit shows negligible catalytic activity in hydrogenation, highlighting ligand-dependent reactivity .
Cyclization Reactions
- Rh₂(COOCF₃)₄ efficiently catalyzes the cyclization of α-diazo-β-ketoesters to oxepanones at room temperature, whereas Rh₂(OAc)₄ requires elevated temperatures .
Biological Activity
Rhodium(II) trifluoroacetate dimer (Rh(OC(CF)), a compound known for its catalytic properties in organic synthesis, has garnered attention in recent years for its potential biological activity. This article explores the compound's mechanisms, biochemical interactions, and relevant research findings.
Chemical Structure : this compound is characterized by its dimeric structure where two rhodium centers are coordinated with four trifluoroacetate ligands. The compound is a green solid and is known to act primarily as a catalyst.
Mechanism of Action :
- Target of Action : The compound predominantly interacts with classical Lewis bases, forming adducts that can influence various biochemical pathways.
- Mode of Action : It binds at the axial positions of the Rhodium(II) centers, facilitating the formation of stable adducts with Lewis bases in a 2:1 stoichiometry .
- Biochemical Pathways : The catalytic activity of this compound is utilized in synthesizing organic compounds, including pharmaceuticals .
Biological Activity and Applications
Research indicates that this compound may have several biological applications due to its unique reactivity:
- Catalysis in Organic Synthesis : Its role as a catalyst in cyclopropanation reactions using diazo compounds demonstrates its utility in creating complex organic structures .
- Pharmaceutical Development : The compound's ability to facilitate reactions that lead to biologically active molecules positions it as a valuable tool in medicinal chemistry .
Toxicity and Environmental Impact
While the compound shows promise in various applications, understanding its toxicity is crucial. Studies have indicated that trifluoroacetate (TFA), a byproduct of this compound, can exhibit toxicity towards aquatic organisms at high concentrations. For instance, acute toxicity tests revealed no effects on Daphnia magna at concentrations up to 1200 mg/L, but lower concentrations showed significant effects on algal species .
Case Studies and Research Findings
Several studies have explored the biological activity and synthesis of this compound:
- Synthesis and Catalytic Activity :
- Reactivity with Lewis Bases :
- NMR Studies :
Comparative Analysis
To better understand the unique properties of this compound, a comparison with similar compounds can be made:
Compound | Structure | Reactivity with Weak Bases | Applications |
---|---|---|---|
This compound | High | Organic synthesis, pharmaceuticals | |
Rhodium(II) acetate dimer | Moderate | Organic synthesis | |
Rhodium(II) heptafluorobutyrate dimer | Moderate | Specialty reactions |
Q & A
Q. Basic: What are the common synthetic applications of Rhodium(II) trifluoroacetate dimer (Rh₂(TFA)₄) in organic chemistry?
Rh₂(TFA)₄ is widely used as a catalyst in cyclization and cyclopropanation reactions. For example, it facilitates the cyclization of α-diazo-ω-hydroxy-β-ketoesters into oxepanones under mild conditions . The catalytic efficiency is attributed to its ability to stabilize carbene intermediates. Methodologically, reactions typically use 1-5 mol% catalyst in dichloromethane or hexanes at room temperature. Product isolation often involves crystallization from solvent mixtures (e.g., dichloromethane-hexanes) .
Q. Basic: How is the Rh-Rh bond structure in Rh₂(TFA)₄ characterized, and what are its implications for reactivity?
The Rh-Rh bond length in Rh₂(TFA)₄ is experimentally determined to be ~2.409–2.415 Å, as confirmed by X-ray crystallography and computational studies. This short bond length enhances stability and facilitates electron transfer during catalysis. Density functional theory (DFT) calculations using scalar relativistic pseudopotentials (e.g., Christiansen-Ermler RECP) accurately predict this bond length, aligning with crystallographic data from 59 dirhodium structures .
Property | Value | Method |
---|---|---|
Rh-Rh bond length | 2.409–2.415 Å | X-ray crystallography/DFT |
Crystal structure | Blue-green powder | Single-crystal XRD |
Q. Basic: What safety protocols are recommended for handling Rh₂(TFA)₄ in laboratory settings?
Rh₂(TFA)₄ is hygroscopic and degrades over time, requiring storage in airtight containers under inert gas (e.g., N₂ or Ar) at –20°C . Personal protective equipment (PPE) such as nitrile gloves, lab coats, and fume hoods are mandatory. Spills should be neutralized with inert adsorbents (e.g., vermiculite) and disposed of as hazardous waste. Prolonged exposure risks include respiratory irritation and metal toxicity .
Q. Advanced: How does heterogenization of Rh₂(TFA)₄ on mesoporous supports affect catalytic performance?
Heterogenization on SBA-15 (a mesoporous silica) functionalized with amine/carboxyl groups improves recyclability and reduces metal leaching. Binding geometry is analyzed via ¹³C/¹⁵N CP MAS and ¹⁹F MAS NMR, which confirm coordination through carboxylate groups. Catalytic activity in styrene cyclopropanation with ethyl diazoacetate (EDA) shows >90% yield over three cycles, with <2% Rh leaching .
Support | Functional Group | Cycles | Yield | Leaching |
---|---|---|---|---|
SBA-15 | Amine/carboxyl | 3 | >90% | <2% |
Q. Advanced: What mechanistic insights explain Rh₂(TFA)₄’s selectivity in asymmetric catalysis?
The dimer’s axial ligands (trifluoroacetate) modulate steric and electronic effects. For example, in cyclopropanation, the Rh₂ core stabilizes carbene intermediates, while trifluoroacetate’s electron-withdrawing nature enhances electrophilicity at the carbene center. Stereoselectivity arises from chiral auxiliaries or supported chiral ligands (e.g., BINOL derivatives), achieving enantiomeric excess (ee) >80% in some cases .
Q. Advanced: How do computational studies aid in predicting Rh₂(TFA)₄’s reactivity with Lewis bases?
DFT and relativistic pseudopotential calculations reveal that Rh₂(TFA)₄ undergoes Rh-Rh bond cleavage upon reaction with strong Lewis bases (e.g., phosphorus donors). For weaker bases (e.g., pyridine), 4:1 adducts form without bond cleavage. These studies guide ligand selection for tuning catalytic activity .
Q. Advanced: What are the environmental implications of trifluoroacetate byproducts from Rh₂(TFA)₄ degradation?
Trifluoroacetic acid (TFA), a degradation byproduct, is highly persistent in aquatic systems. Analytical methods like GC-MS and ion chromatography detect TFA at ppm levels. Remediation strategies include advanced oxidation processes (AOPs) or adsorption onto activated carbon .
Q. Basic: Which spectroscopic techniques are used to characterize Rh₂(TFA)₄ and its intermediates?
- ¹⁹F NMR : Tracks trifluoroacetate ligand exchange (δ = –75 to –80 ppm).
- UV-Vis : Monitors carbene intermediate formation (λ = 450–500 nm).
- XRD : Confirms crystal structure and Rh-Rh bond metrics .
Q. Advanced: How does solvent polarity influence Rh₂(TFA)₄’s catalytic efficiency in hydrogenation?
In polar solvents (e.g., DMF), solvation stabilizes the dirhodium core, reducing aggregation. This enhances hydrogenation rates for nitroarenes (e.g., o-nitrophenol) by up to 50% compared to nonpolar solvents (e.g., toluene). Kinetic studies using in situ IR spectroscopy validate solvent-dependent turnover frequencies (TOFs) .
Q. Basic: What are the synthetic alternatives to Rh₂(TFA)₄ for dirhodium-catalyzed reactions?
Rhodium(II) acetate (Rh₂(OAc)₄) and Rh₂(octanoate)₄ are common alternatives. However, Rh₂(TFA)₄’s superior solubility in halogenated solvents and higher electrophilicity make it preferable for reactions requiring rigorous anhydrous conditions .
Properties
CAS No. |
31126-95-1 |
---|---|
Molecular Formula |
C8F12O8Rh2 |
Molecular Weight |
657.87 g/mol |
IUPAC Name |
rhodium(2+);2,2,2-trifluoroacetate |
InChI |
InChI=1S/4C2HF3O2.2Rh/c4*3-2(4,5)1(6)7;;/h4*(H,6,7);;/q;;;;2*+2/p-4 |
InChI Key |
PQEXTYUESSEHKW-UHFFFAOYSA-J |
SMILES |
C(=O)(C(F)(F)F)O.C(=O)(C(F)(F)F)O.C(=O)(C(F)(F)F)O.C(=O)(C(F)(F)F)O.[Rh].[Rh] |
Canonical SMILES |
C(=O)(C(F)(F)F)[O-].C(=O)(C(F)(F)F)[O-].C(=O)(C(F)(F)F)[O-].C(=O)(C(F)(F)F)[O-].[Rh+2].[Rh+2] |
Origin of Product |
United States |
Retrosynthesis Analysis
AI-Powered Synthesis Planning: Our tool employs the Template_relevance Pistachio, Template_relevance Bkms_metabolic, Template_relevance Pistachio_ringbreaker, Template_relevance Reaxys, Template_relevance Reaxys_biocatalysis model, leveraging a vast database of chemical reactions to predict feasible synthetic routes.
One-Step Synthesis Focus: Specifically designed for one-step synthesis, it provides concise and direct routes for your target compounds, streamlining the synthesis process.
Accurate Predictions: Utilizing the extensive PISTACHIO, BKMS_METABOLIC, PISTACHIO_RINGBREAKER, REAXYS, REAXYS_BIOCATALYSIS database, our tool offers high-accuracy predictions, reflecting the latest in chemical research and data.
Strategy Settings
Precursor scoring | Relevance Heuristic |
---|---|
Min. plausibility | 0.01 |
Model | Template_relevance |
Template Set | Pistachio/Bkms_metabolic/Pistachio_ringbreaker/Reaxys/Reaxys_biocatalysis |
Top-N result to add to graph | 6 |
Feasible Synthetic Routes
Disclaimer and Information on In-Vitro Research Products
Please be aware that all articles and product information presented on BenchChem are intended solely for informational purposes. The products available for purchase on BenchChem are specifically designed for in-vitro studies, which are conducted outside of living organisms. In-vitro studies, derived from the Latin term "in glass," involve experiments performed in controlled laboratory settings using cells or tissues. It is important to note that these products are not categorized as medicines or drugs, and they have not received approval from the FDA for the prevention, treatment, or cure of any medical condition, ailment, or disease. We must emphasize that any form of bodily introduction of these products into humans or animals is strictly prohibited by law. It is essential to adhere to these guidelines to ensure compliance with legal and ethical standards in research and experimentation.