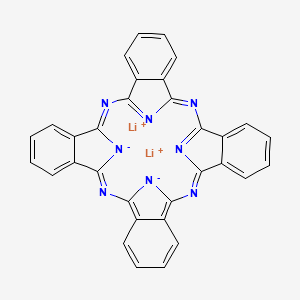
Dilithium phthalocyanine
Overview
Description
Dilithium phthalocyanine (Li₂Pc, CAS 25510-41-2) is a planar macrocyclic compound with two lithium ions coordinated within its 18π-electron aromatic structure. Its molecular formula is C₃₂H₁₆Li₂N₈, with a molecular weight of 526.42 g/mol . Li₂Pc exhibits high thermal stability (stable up to ~300°C) and solubility in polar solvents like acetone and ethanol, but insolubility in non-polar solvents such as chloroform or hexane . Key applications include:
- Electronics: Organic semiconductors, OLEDs .
- Photovoltaics: Photosensitizer in solar cells for enhanced energy conversion .
- Catalysis: Efficient catalyst in green chemistry processes .
- Sensors: High-sensitivity gas and chemical detection .
Its electronic spectrum in acetone shows absorption bands at 370 nm (ε = 24,800), 596 nm (ε = 17,300), and 655 nm (ε = 11,100) . Safety data indicate toxicity (Hazard Code T) with risks including reproductive toxicity (H360) .
Preparation Methods
Synthetic Routes and Reaction Conditions
Direct Metallation of Phthalocyanine Precursors
The most common preparation method involves the metallation of phthalocyanine precursors, such as phthalonitrile, with lithium metal or lithium salts under inert atmosphere conditions to prevent oxidation. The reaction typically occurs in aprotic solvents like acetonitrile or dimethyl sulfoxide (DMSO), which dissolve the reactants and support the formation of the dilithium phthalocyanine complex.
-
- Reactants: Phthalonitrile and lithium metal
- Solvent: Acetonitrile or DMSO
- Atmosphere: Inert (nitrogen or argon)
- Temperature: Room temperature or reflux depending on solvent and scale
- Duration: Several hours to overnight
Purification: The product is purified by recrystallization or chromatographic techniques to remove impurities and unreacted starting materials.
Reaction Mechanism and Phase Control
- The synthesis proceeds via the cyclization of phthalonitrile units coordinated to lithium ions, forming the macrocyclic phthalocyanine ring with lithium centers.
- The γ-phase of lithium phthalocyanine is preferred for its oxygen sensitivity and electronic properties. This phase is selectively obtained by controlling the synthesis conditions, especially avoiding high-temperature treatments that convert the α-phase to the β-phase.
- Electrochemical synthesis allows for precise control of the oxidation state and phase purity by adjusting the applied potential and reaction time.
Comparative Analysis of Preparation Methods
Method | Advantages | Limitations | Typical Applications |
---|---|---|---|
Direct Metallation | Simple, scalable, uses common reagents | Requires inert atmosphere, sensitive to moisture | Industrial scale, bulk synthesis |
Electrochemical Synthesis | High purity, phase control, fine crystal growth | Requires electrochemical setup, longer time | Research-grade materials, sensor applications |
Research Findings and Data
- Crystallographic and Morphological Data: SEM imaging confirms needle-like crystals with average lengths of ~2 µm and widths of ~0.4 µm, which is consistent with literature reports for γ-LiPc crystals.
- Spectroscopic Characterization: EPR spectroscopy is extensively used to characterize the paramagnetic centers in Li2Pc, with linewidths sensitive to oxygen partial pressure, indicating high purity and phase specificity.
- Reaction Yields: Direct metallation methods report yields up to 70% depending on solvent and reaction time, with careful exclusion of moisture to prevent side reactions.
Summary Table of Preparation Parameters
Preparation Method | Reactants | Solvent | Conditions | Product Phase | Purification Method | Yield (%) |
---|---|---|---|---|---|---|
Direct Metallation | Phthalonitrile + Li metal | Acetonitrile/DMSO | Inert atmosphere, reflux/RT | γ-phase | Recrystallization/Chromatography | Up to 70 |
Electrochemical Synthesis | This compound salt | Dry acetonitrile | 0.05–0.75 V, 12 h, RT | γ-phase | Washing and crystal collection | Not specified |
Chemical Reactions Analysis
Types of Reactions: Dilithium phthalocyanine undergoes various chemical reactions, including:
Oxidation: The compound can be oxidized to form different oxidation states, which are useful in various catalytic processes.
Reduction: It can be reduced to form lower oxidation states, which are often used in electronic applications.
Substitution: The compound can undergo substitution reactions where one or more of its hydrogen atoms are replaced by other atoms or groups.
Common Reagents and Conditions:
Oxidation: Common oxidizing agents include potassium permanganate and hydrogen peroxide.
Reduction: Reducing agents such as lithium aluminum hydride and sodium borohydride are frequently used.
Substitution: Substitution reactions often involve halogens or other electrophilic reagents under controlled conditions.
Major Products Formed: The major products formed from these reactions depend on the specific reagents and conditions used. For example, oxidation may yield various oxidized forms of the compound, while substitution reactions can produce a wide range of derivatives .
Scientific Research Applications
Electronic Applications
1.1 Organic Semiconductors
Dilithium phthalocyanine has been extensively studied for its use in organic semiconductors. Thin films of LiPc exhibit semiconductor behavior, making them suitable for applications in:
- Organic Field-Effect Transistors (OFETs)
- Organic Light Emitting Diodes (OLEDs)
- Solar Cells
A study characterized LiPc thin films grown on quartz and silicon substrates, revealing high electrical conductivities and thermal stability, which are crucial for electronic applications . The films were analyzed using techniques such as Fourier Transform Infrared (FTIR) spectroscopy and Atomic Force Microscopy (AFM), demonstrating the material's potential in electronic devices.
Application Type | Key Findings |
---|---|
OFETs | High charge carrier mobility observed. |
OLEDs | Enhanced light emission efficiency due to optimal film morphology. |
Solar Cells | Improved power conversion efficiency with LiPc-based materials. |
1.2 Electrochemical Applications
LiPc has also been investigated as an electrolyte additive in lithium metal batteries. It regulates ion solvation and transport, contributing to dendrite-free lithium anodes, which are critical for enhancing battery safety and longevity .
Photonic Applications
This compound is utilized in photonic devices due to its strong light absorption properties. The compound's ability to form stable thin films allows for its use in:
- Xerographic Photoreceptors
- Chemical Sensors
- Imaging Systems
The stability of LiPc films under various conditions makes them ideal for these applications. Research indicates that these films maintain their integrity and performance over time, making them suitable for long-term use in photonic devices .
Catalytic Applications
Recent studies have highlighted the role of this compound in catalysis. When integrated with carbon nanofibers, it serves as a catalyst for various chemical reactions, enhancing efficiency and selectivity . This application is particularly relevant in green chemistry, where the reduction of hazardous substances is a priority.
Biomedical Applications
Emerging research suggests potential biomedical applications for this compound, particularly in drug delivery systems. Its properties allow for encapsulation of therapeutic agents, providing controlled release mechanisms that are essential for treating chronic diseases .
Biomedical Application | Key Benefits |
---|---|
Drug Delivery | Controlled release and reduced toxicity of encapsulated drugs. |
Imaging | Potential use as a contrast agent due to its optical properties. |
Case Studies
Case Study 1: Organic Electronics
A study demonstrated the synthesis of amorphous molecular semiconductors from LiPc combined with bidentate ligands. The resulting thin films exhibited significant electrical conductivity and favorable optical properties, affirming their utility in organic electronics .
Case Study 2: Battery Technology
Research on LiPc as an electrolyte additive showed that it effectively regulates ion transport in lithium metal batteries, leading to enhanced performance metrics such as cycle stability and capacity retention over extended periods .
Mechanism of Action
The mechanism of action of Dilithium phthalocyanine involves its interaction with various molecular targets and pathways. The compound can act as a photosensitizer, generating reactive oxygen species upon exposure to light, which can induce cell death in targeted cells. This property is particularly useful in photodynamic therapy for cancer treatment .
Comparison with Similar Compounds
Comparison with Similar Phthalocyanine Compounds
Phthalocyanines (Pcs) are a versatile class of macrocycles with tunable properties based on central metals and substituents. Below, Li₂Pc is compared to other Pcs in terms of structure, properties, and applications.
Structural and Physical Properties
Key Observations :
- Li₂Pc’s solubility in polar solvents contrasts with CuPc’s insolubility, making Li₂Pc preferable for solution-based processing in electronics .
- CuPc and ZnPc exhibit higher thermal stability, but Li₂Pc’s stability is sufficient for sublimation-based applications .
Electrochemical and Optical Properties
Nonlinear Optical (NLO) Performance
Key Observations :
- Li₂Pc’s NLO properties are understudied compared to ZnPc dimers, which show superior two-photon absorption .
- CuPc derivatives are preferred in imaging due to lower cost and established synthesis .
Key Observations :
- Li₂Pc’s dominance in electronics/photovoltaics is driven by its conductivity and compatibility with thin-film technologies .
- CuPc remains the industry standard for blue/green pigments but lacks versatility in high-tech applications .
Key Observations :
- Li₂Pc’s toxicity necessitates stringent handling compared to ZnPc, limiting its use in biomedical fields .
Q & A
Basic Research Questions
Q. What are the optimal synthesis methods for dilithium phthalocyanine to ensure high purity and structural integrity?
- This compound synthesis typically involves metallation of phthalocyanine precursors with lithium salts under inert conditions. Key parameters include stoichiometric control of lithium ions and reaction temperature (e.g., 80–120°C). Post-synthesis purification via Soxhlet extraction with methanol or ethanol removes unreacted precursors. Structural verification requires XRD for crystallinity and FTIR to confirm Li-N coordination . Purity (>93%) can be quantified via elemental analysis and UV-Vis spectroscopy .
Q. How can researchers characterize the optical properties of this compound thin films?
- Optical bandgap determination uses UV-Vis spectroscopy combined with Tauc and Cody models. For amorphous films, the Tauc model applies to direct transitions, while the Cody model accounts for disorder-induced absorption tails. A slope analysis of (αhν)^(1/2) vs. hν (Cody) or (αhν)^2 vs. hν (Tauc) reveals bandgap values. Discrepancies between models highlight film heterogeneity, necessitating AFM or SEM for morphological validation .
Q. What spectroscopic techniques are critical for confirming lithium coordination in this compound?
- X-ray photoelectron spectroscopy (XPS) identifies Li 1s binding energy shifts (typically ~55–56 eV) indicative of Li-N bonding. FTIR spectroscopy detects shifts in the phthalocyanine macrocycle’s vibrational modes (e.g., C-N stretching at ~1330 cm⁻¹) upon lithiation. Solid-state NMR (⁷Li) further resolves lithium’s local electronic environment .
Advanced Research Questions
Q. How can experimental design optimize this compound’s electrocatalytic activity for hydrogen evolution reactions (HER)?
- Bimetallic or trimetallic doping (e.g., Ni or Co incorporation) enhances HER activity by modifying the phthalocyanine’s electronic structure. Electrochemical impedance spectroscopy (EIS) and Tafel analysis quantify charge-transfer resistance and overpotential. Functionalization temperatures (300–500°C) and precursor ratios (e.g., Ni:Li molar ratios) are systematically varied, with activity benchmarks measured via rotating disk electrode (RDE) voltammetry in 1 M KOH .
Q. What methodologies resolve contradictions in charge transport data for this compound-based devices?
- Discrepancies in conductivity measurements (e.g., DC vs. AC methods) arise from grain boundary effects in polycrystalline films. Temperature-dependent DC conductivity studies (25–150°C) distinguish intrinsic semiconductor behavior from extrinsic factors like impurities. For thin films, Hall effect measurements provide carrier mobility and density, while Mott-Schottky analysis probes interfacial charge transfer in heterostructures .
Q. How does this compound function as a Li-ion transport regulator in lithium-metal batteries?
- As an electrolyte additive, this compound solvates Li⁺ ions via its macrocyclic cavity, reducing anion mobility and suppressing dendrite formation. Symmetric Li||Li cell cycling (0.5–2 mA/cm²) quantifies overpotential stability. Raman spectroscopy tracks Li⁺-solvent vs. Li⁺-phthalocyanine interactions, while Arrhenius plots of ionic conductivity reveal activation energy changes .
Q. What strategies mitigate aggregation-induced quenching in this compound-based optoelectronic devices?
- Nanostructuring (e.g., embedding in mesoporous TiO₂) or covalent functionalization with bulky substituents (e.g., tert-butyl groups) reduces π-π stacking. Time-resolved photoluminescence (TRPL) measures excited-state lifetimes, while atomic layer deposition (ALD) creates protective coatings to minimize environmental degradation .
Q. Methodological Guidance
Q. How should researchers design experiments to study this compound’s stability under operational conditions?
- Accelerated aging tests (e.g., 85°C/85% humidity for 500 hours) assess thermal and moisture stability. In situ XRD monitors structural changes, while XPS post-testing identifies oxidation products (e.g., Li₂CO₃). For electrochemical stability, cyclic voltammetry (100+ cycles) evaluates redox peak retention .
Q. What statistical approaches are recommended for analyzing variability in this compound’s optical data?
- Multivariate analysis (e.g., principal component analysis, PCA) isolates experimental variables (e.g., film thickness, doping concentration). Error bars in Tauc/Cody plots are derived from triplicate measurements, and bootstrapping validates optical gap uncertainties .
Q. Data Interpretation and Reproducibility
Q. How can researchers address batch-to-batch variability in this compound synthesis?
- Implement a quality-by-design (QbD) framework: Critical process parameters (CPPs) like reaction time and solvent purity are controlled using design of experiments (DoE). Batch consistency is verified via HPLC purity profiles and comparative XRD/FTIR spectra .
Q. What protocols ensure reproducibility in this compound thin-film deposition?
Properties
CAS No. |
25510-41-2 |
---|---|
Molecular Formula |
C32H18LiN8 |
Molecular Weight |
521.5 g/mol |
IUPAC Name |
dilithium;2,11,20,29,37,39-hexaza-38,40-diazanidanonacyclo[28.6.1.13,10.112,19.121,28.04,9.013,18.022,27.031,36]tetraconta-1,3,5,7,9,11,13,15,17,19(39),20,22,24,26,28,30(37),31,33,35-nonadecaene |
InChI |
InChI=1S/C32H18N8.Li/c1-2-10-18-17(9-1)25-33-26(18)38-28-21-13-5-6-14-22(21)30(35-28)40-32-24-16-8-7-15-23(24)31(36-32)39-29-20-12-4-3-11-19(20)27(34-29)37-25;/h1-16H,(H2,33,34,35,36,37,38,39,40); |
InChI Key |
CNULBOCCFMPDKX-UHFFFAOYSA-N |
SMILES |
[Li+].[Li+].C1=CC=C2C(=C1)C3=NC4=C5C=CC=CC5=C([N-]4)N=C6C7=CC=CC=C7C(=N6)N=C8C9=CC=CC=C9C(=N8)N=C2[N-]3 |
Canonical SMILES |
[Li].C1=CC=C2C(=C1)C3=NC2=NC4=NC(=NC5=NC(=NC6=C7C=CC=CC7=C(N6)N3)C8=CC=CC=C85)C9=CC=CC=C94 |
Key on ui other cas no. |
25510-41-2 |
Origin of Product |
United States |
Retrosynthesis Analysis
AI-Powered Synthesis Planning: Our tool employs the Template_relevance Pistachio, Template_relevance Bkms_metabolic, Template_relevance Pistachio_ringbreaker, Template_relevance Reaxys, Template_relevance Reaxys_biocatalysis model, leveraging a vast database of chemical reactions to predict feasible synthetic routes.
One-Step Synthesis Focus: Specifically designed for one-step synthesis, it provides concise and direct routes for your target compounds, streamlining the synthesis process.
Accurate Predictions: Utilizing the extensive PISTACHIO, BKMS_METABOLIC, PISTACHIO_RINGBREAKER, REAXYS, REAXYS_BIOCATALYSIS database, our tool offers high-accuracy predictions, reflecting the latest in chemical research and data.
Strategy Settings
Precursor scoring | Relevance Heuristic |
---|---|
Min. plausibility | 0.01 |
Model | Template_relevance |
Template Set | Pistachio/Bkms_metabolic/Pistachio_ringbreaker/Reaxys/Reaxys_biocatalysis |
Top-N result to add to graph | 6 |
Feasible Synthetic Routes
Disclaimer and Information on In-Vitro Research Products
Please be aware that all articles and product information presented on BenchChem are intended solely for informational purposes. The products available for purchase on BenchChem are specifically designed for in-vitro studies, which are conducted outside of living organisms. In-vitro studies, derived from the Latin term "in glass," involve experiments performed in controlled laboratory settings using cells or tissues. It is important to note that these products are not categorized as medicines or drugs, and they have not received approval from the FDA for the prevention, treatment, or cure of any medical condition, ailment, or disease. We must emphasize that any form of bodily introduction of these products into humans or animals is strictly prohibited by law. It is essential to adhere to these guidelines to ensure compliance with legal and ethical standards in research and experimentation.