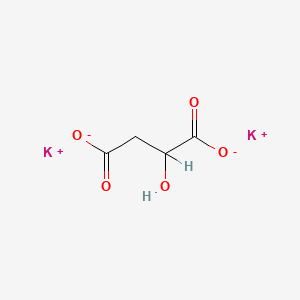
Dipotassium malate
Overview
Description
Dipotassium malate is a chemical compound with the formula ( \text{K}_2(\text{C}_4\text{H}_4\text{O}_5) ). It is the potassium salt of malic acid, which is a naturally occurring substance found in many fruits and vegetables. This compound is commonly used as a food additive, where it serves as an acidity regulator, flavor enhancer, and antioxidant. It is also involved in various biological processes, including the transport of nitrate in plants .
Mechanism of Action
Target of Action
Dipotassium malate, the potassium salt of malic acid, primarily targets the biochemical pathways in plants and algae . It plays a crucial role in the transport of nitrate from the roots of a plant to the leaves .
Mode of Action
This compound acts as a transporter molecule in plants. It carries nitrate from the roots to the leaves of the plant. At the root, this compound oxidizes to potassium carbonate, then is converted to potassium nitrate by soil nitrate and transported back to the leaves .
Biochemical Pathways
This compound is involved in the malate-aspartate shuttle, a biochemical system for translocating electrons produced during glycolysis across the semipermeable inner membrane of the mitochondrion for oxidative phosphorylation in eukaryotes . It also plays a role in the tricarboxylic acid (TCA), C4-dicarboxylic acid, and glyoxylate cycles in plants and algae .
Pharmacokinetics
It is known that it is highly water-soluble , which may influence its bioavailability and distribution within the organism.
Result of Action
The primary result of this compound’s action is the successful transport of nitrate from the roots to the leaves of a plant. This process is essential for the plant’s nutrient uptake and overall health .
Action Environment
The action of this compound is influenced by environmental factors such as soil nitrate levels and the presence of water, given its role in nitrate transport and its water solubility
Biochemical Analysis
Biochemical Properties
Dipotassium malate is involved in several biochemical reactions, primarily due to its role as an intermediate in the citric acid cycle (Krebs cycle). This compound interacts with various enzymes, including malate dehydrogenase, which catalyzes the conversion of malate to oxaloacetate. Additionally, this compound interacts with fumarase, facilitating the hydration of fumarate to malate. These interactions are crucial for the production of energy in the form of adenosine triphosphate (ATP) within cells .
Cellular Effects
This compound influences various cellular processes, including cell signaling pathways, gene expression, and cellular metabolism. It plays a role in the regulation of intracellular pH and osmotic balance. By participating in the citric acid cycle, this compound contributes to the production of ATP, which is essential for cellular energy. Furthermore, it affects the expression of genes involved in metabolic pathways, thereby influencing overall cellular function .
Molecular Mechanism
At the molecular level, this compound exerts its effects through several mechanisms. It binds to specific enzymes, such as malate dehydrogenase, and facilitates the conversion of malate to oxaloacetate. This reaction is coupled with the reduction of nicotinamide adenine dinucleotide (NAD+) to NADH, which is then used in the electron transport chain to generate ATP. Additionally, this compound can act as an allosteric regulator of certain enzymes, modulating their activity and influencing metabolic flux .
Temporal Effects in Laboratory Settings
In laboratory settings, the effects of this compound can vary over time. The stability of this compound is influenced by factors such as temperature, pH, and the presence of other compounds. Over time, this compound may degrade, leading to changes in its biochemical activity. Long-term studies have shown that this compound can have sustained effects on cellular function, particularly in terms of energy production and metabolic regulation .
Dosage Effects in Animal Models
The effects of this compound in animal models are dose-dependent. At low doses, this compound can enhance metabolic activity and improve energy production. At high doses, it may lead to adverse effects such as metabolic imbalances and toxicity. Studies have shown that there is a threshold beyond which the beneficial effects of this compound are outweighed by its potential toxicity .
Metabolic Pathways
This compound is involved in several metabolic pathways, including the citric acid cycle, glyoxylate cycle, and malate-aspartate shuttle. In the citric acid cycle, it is converted to oxaloacetate by malate dehydrogenase. In the glyoxylate cycle, this compound is converted to glyoxylate and acetyl-CoA. The malate-aspartate shuttle facilitates the transfer of reducing equivalents across the mitochondrial membrane, playing a crucial role in cellular respiration .
Transport and Distribution
Within cells, this compound is transported and distributed through various mechanisms. It can be transported across cellular membranes by specific transporters, such as dicarboxylate transporters. Once inside the cell, this compound can be localized to different cellular compartments, including the cytosol and mitochondria. Its distribution is influenced by factors such as cellular energy demand and metabolic state .
Subcellular Localization
This compound is primarily localized in the cytosol and mitochondria. In the cytosol, it participates in metabolic pathways such as glycolysis and the citric acid cycle. In the mitochondria, this compound plays a crucial role in the production of ATP through oxidative phosphorylation. The subcellular localization of this compound is regulated by specific targeting signals and post-translational modifications that direct it to the appropriate cellular compartments .
Preparation Methods
Synthetic Routes and Reaction Conditions
Dipotassium malate can be synthesized by reacting malic acid with potassium carbonate. The reaction typically occurs in an aqueous solution at a temperature range of 50 to 80 degrees Celsius. The reaction is completed within 0.5 to 1 hour, after which the mixture undergoes solid-liquid separation to obtain the filtrate. The filtrate is then concentrated to yield this compound .
Industrial Production Methods
In industrial settings, the preparation of this compound involves similar steps but on a larger scale. The process includes mixing malic acid with potassium carbonate in a controlled environment to ensure optimal reaction conditions. The resulting product is then purified, dried, and packaged for various applications .
Chemical Reactions Analysis
Types of Reactions
Dipotassium malate undergoes several types of chemical reactions, including:
Oxidation: this compound can be oxidized to form potassium carbonate and other by-products.
Reduction: It can be reduced under specific conditions to yield different potassium salts.
Substitution: The compound can participate in substitution reactions where one or more of its functional groups are replaced by other atoms or groups.
Common Reagents and Conditions
Oxidation: Common oxidizing agents include potassium permanganate and hydrogen peroxide.
Reduction: Reducing agents such as sodium borohydride can be used.
Substitution: Various acids and bases can facilitate substitution reactions.
Major Products
Oxidation: Potassium carbonate and water.
Reduction: Potassium salts and water.
Substitution: Various substituted malate compounds depending on the reagents used.
Scientific Research Applications
Dipotassium malate has a wide range of applications in scientific research:
Chemistry: Used as a reagent in various chemical reactions and as a buffering agent.
Biology: Plays a role in the transport of nitrate in plants and is used in studies related to plant physiology.
Medicine: Investigated for its potential benefits in improving energy levels, bone health, and cardiovascular health.
Industry: Utilized as a food additive for its acidity regulation, flavor enhancement, and antioxidant properties .
Comparison with Similar Compounds
Similar Compounds
Monopotassium malate: Another potassium salt of malic acid, used similarly as a food additive.
Potassium hydrogen malate: Used in food and pharmaceutical industries.
Calcium malate: Used as a calcium supplement and in food applications.
Uniqueness
Dipotassium malate is unique due to its specific role in nitrate transport in plants and its dual functionality as both an acidity regulator and antioxidant in food applications. Its ability to participate in various chemical reactions also makes it versatile for scientific research .
Properties
CAS No. |
585-09-1 |
---|---|
Molecular Formula |
C4H6KO5 |
Molecular Weight |
173.19 g/mol |
IUPAC Name |
dipotassium;2-hydroxybutanedioate |
InChI |
InChI=1S/C4H6O5.K/c5-2(4(8)9)1-3(6)7;/h2,5H,1H2,(H,6,7)(H,8,9); |
InChI Key |
VXUDZHAEAWUUPX-UHFFFAOYSA-N |
SMILES |
C(C(C(=O)[O-])O)C(=O)[O-].[K+].[K+] |
Canonical SMILES |
C(C(C(=O)O)O)C(=O)O.[K] |
Key on ui other cas no. |
585-09-1 |
physical_description |
Colourless or almost colourless aqueous solution |
Related CAS |
4675-64-3 |
Origin of Product |
United States |
Retrosynthesis Analysis
AI-Powered Synthesis Planning: Our tool employs the Template_relevance Pistachio, Template_relevance Bkms_metabolic, Template_relevance Pistachio_ringbreaker, Template_relevance Reaxys, Template_relevance Reaxys_biocatalysis model, leveraging a vast database of chemical reactions to predict feasible synthetic routes.
One-Step Synthesis Focus: Specifically designed for one-step synthesis, it provides concise and direct routes for your target compounds, streamlining the synthesis process.
Accurate Predictions: Utilizing the extensive PISTACHIO, BKMS_METABOLIC, PISTACHIO_RINGBREAKER, REAXYS, REAXYS_BIOCATALYSIS database, our tool offers high-accuracy predictions, reflecting the latest in chemical research and data.
Strategy Settings
Precursor scoring | Relevance Heuristic |
---|---|
Min. plausibility | 0.01 |
Model | Template_relevance |
Template Set | Pistachio/Bkms_metabolic/Pistachio_ringbreaker/Reaxys/Reaxys_biocatalysis |
Top-N result to add to graph | 6 |
Feasible Synthetic Routes
Q1: What is the structural characterization of dipotassium malate, and how was this information used in scientific research?
A1: this compound is an organic salt derived from malic acid, a dicarboxylic acid found naturally in fruits like apples. While the provided abstracts do not detail the exact molecular formula and weight of this compound, they highlight its use in nuclear magnetic resonance (NMR) studies. One study investigated the this compound's ABX proton spin system using NMR double resonance techniques []. Researchers used this spectroscopic data, alongside density matrix calculations, to determine the relative signs of coupling constants within the molecule. This type of analysis provides valuable insights into the molecule's three-dimensional structure and the interactions between its atoms.
Q2: Can you elaborate on the specific application of this compound in electromembrane processes as mentioned in one of the research papers?
A2: One of the research papers focuses on the conversion of this compound to malic acid using electromembrane processes []. While the abstract doesn't provide specific details about the process, it suggests that this compound can be used as a starting material for the sustainable production of malic acid. Electromembrane processes, known for their efficiency and environmental friendliness, likely utilize ion exchange membranes to separate and concentrate malic acid from the dipotassium salt. This approach could offer advantages over traditional chemical conversion methods.
Disclaimer and Information on In-Vitro Research Products
Please be aware that all articles and product information presented on BenchChem are intended solely for informational purposes. The products available for purchase on BenchChem are specifically designed for in-vitro studies, which are conducted outside of living organisms. In-vitro studies, derived from the Latin term "in glass," involve experiments performed in controlled laboratory settings using cells or tissues. It is important to note that these products are not categorized as medicines or drugs, and they have not received approval from the FDA for the prevention, treatment, or cure of any medical condition, ailment, or disease. We must emphasize that any form of bodily introduction of these products into humans or animals is strictly prohibited by law. It is essential to adhere to these guidelines to ensure compliance with legal and ethical standards in research and experimentation.