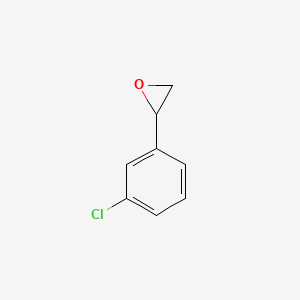
2-(3-Chlorophenyl)oxirane
Overview
Description
2-(3-Chlorophenyl)oxirane: is an organic compound characterized by the presence of an oxirane ring (a three-membered cyclic ether) attached to a 3-chlorophenyl group
Preparation Methods
Synthetic Routes and Reaction Conditions: The synthesis of 2-(3-Chlorophenyl)oxirane typically involves the epoxidation of 3-chlorostyrene. One common method is the reaction of 3-chlorostyrene with a peracid, such as m-chloroperoxybenzoic acid (mCPBA), under mild conditions. The reaction proceeds as follows:
3-chlorostyrene+mCPBA→this compound+m-chlorobenzoic acid
The reaction is usually carried out in an inert solvent like dichloromethane at room temperature.
Industrial Production Methods: On an industrial scale, the production of this compound can be achieved through the chlorohydrin method. This involves the chlorination of 3-chlorostyrene to form the corresponding chlorohydrin, followed by dehydrochlorination using a base such as sodium hydroxide. The process can be summarized as:
3-chlorostyrene+Cl2→3-chlorostyrene chlorohydrin
3-chlorostyrene chlorohydrin+NaOH→this compound+NaCl+H2O
Chemical Reactions Analysis
Types of Reactions: 2-(3-Chlorophenyl)oxirane undergoes various types of chemical reactions, including:
Nucleophilic Ring-Opening Reactions: The oxirane ring is highly strained and thus susceptible to nucleophilic attack. Common nucleophiles include water, alcohols, and amines, leading to the formation of diols, ethers, and amino alcohols, respectively.
Reduction Reactions: The compound can be reduced to form 3-chlorophenylethanol using reducing agents such as lithium aluminum hydride (LiAlH4).
Substitution Reactions: The chlorine atom on the phenyl ring can undergo nucleophilic substitution reactions, leading to the formation of various substituted phenyl oxiranes.
Common Reagents and Conditions:
Nucleophilic Ring-Opening: Acidic or basic conditions, with nucleophiles such as water, methanol, or ammonia.
Reduction: Lithium aluminum hydride (LiAlH4) in anhydrous ether.
Substitution: Nucleophiles such as sodium methoxide (NaOCH3) in methanol.
Major Products:
Diols: Formed from the hydrolysis of the oxirane ring.
Ethers: Formed from the reaction with alcohols.
Amino Alcohols: Formed from the reaction with amines.
3-Chlorophenylethanol: Formed from the reduction of the oxirane ring.
Scientific Research Applications
2-(3-Chlorophenyl)oxirane has several applications in scientific research:
Organic Synthesis: Used as an intermediate in the synthesis of various organic compounds, including pharmaceuticals and agrochemicals.
Medicinal Chemistry: Investigated for its potential as a building block in the synthesis of biologically active molecules, including anticancer and antimicrobial agents.
Materials Science: Utilized in the preparation of polymers and resins with specific properties.
Biological Studies: Studied for its interactions with biological molecules and potential use in biochemical assays.
Mechanism of Action
The mechanism of action of 2-(3-Chlorophenyl)oxirane primarily involves the reactivity of the oxirane ring. The strained three-membered ring is prone to nucleophilic attack, leading to ring-opening reactions. This reactivity is exploited in various synthetic transformations and biological interactions. The molecular targets and pathways involved depend on the specific application and the nature of the nucleophile or other reactants.
Comparison with Similar Compounds
2-(4-Chlorophenyl)oxirane: Similar structure but with the chlorine atom at the para position.
2-(2-Chlorophenyl)oxirane: Chlorine atom at the ortho position.
2-Phenyl oxirane: Lacks the chlorine substituent.
Comparison:
Reactivity: The position of the chlorine atom affects the reactivity of the oxirane ring. For example, the para-substituted compound may have different steric and electronic effects compared to the meta-substituted 2-(3-Chlorophenyl)oxirane.
Applications: The presence and position of the chlorine atom can influence the compound’s suitability for specific applications, such as in medicinal chemistry or materials science.
Uniqueness: this compound is unique due to its specific substitution pattern, which can lead to distinct reactivity and properties compared to its isomers and analogs.
Properties
IUPAC Name |
2-(3-chlorophenyl)oxirane | |
---|---|---|
Source | PubChem | |
URL | https://pubchem.ncbi.nlm.nih.gov | |
Description | Data deposited in or computed by PubChem | |
InChI |
InChI=1S/C8H7ClO/c9-7-3-1-2-6(4-7)8-5-10-8/h1-4,8H,5H2 | |
Source | PubChem | |
URL | https://pubchem.ncbi.nlm.nih.gov | |
Description | Data deposited in or computed by PubChem | |
InChI Key |
YVMKRPGFBQGEBF-UHFFFAOYSA-N | |
Source | PubChem | |
URL | https://pubchem.ncbi.nlm.nih.gov | |
Description | Data deposited in or computed by PubChem | |
Canonical SMILES |
C1C(O1)C2=CC(=CC=C2)Cl | |
Source | PubChem | |
URL | https://pubchem.ncbi.nlm.nih.gov | |
Description | Data deposited in or computed by PubChem | |
Molecular Formula |
C8H7ClO | |
Source | PubChem | |
URL | https://pubchem.ncbi.nlm.nih.gov | |
Description | Data deposited in or computed by PubChem | |
DSSTOX Substance ID |
DTXSID40942899 | |
Record name | 2-(3-Chlorophenyl)oxirane | |
Source | EPA DSSTox | |
URL | https://comptox.epa.gov/dashboard/DTXSID40942899 | |
Description | DSSTox provides a high quality public chemistry resource for supporting improved predictive toxicology. | |
Molecular Weight |
154.59 g/mol | |
Source | PubChem | |
URL | https://pubchem.ncbi.nlm.nih.gov | |
Description | Data deposited in or computed by PubChem | |
CAS No. |
20697-04-5 | |
Record name | 2-(3-Chlorophenyl)oxirane | |
Source | CAS Common Chemistry | |
URL | https://commonchemistry.cas.org/detail?cas_rn=20697-04-5 | |
Description | CAS Common Chemistry is an open community resource for accessing chemical information. Nearly 500,000 chemical substances from CAS REGISTRY cover areas of community interest, including common and frequently regulated chemicals, and those relevant to high school and undergraduate chemistry classes. This chemical information, curated by our expert scientists, is provided in alignment with our mission as a division of the American Chemical Society. | |
Explanation | The data from CAS Common Chemistry is provided under a CC-BY-NC 4.0 license, unless otherwise stated. | |
Record name | Benzene, 1-chloro-3-(epoxyethyl)- | |
Source | ChemIDplus | |
URL | https://pubchem.ncbi.nlm.nih.gov/substance/?source=chemidplus&sourceid=0020697045 | |
Description | ChemIDplus is a free, web search system that provides access to the structure and nomenclature authority files used for the identification of chemical substances cited in National Library of Medicine (NLM) databases, including the TOXNET system. | |
Record name | 2-(3-Chlorophenyl)oxirane | |
Source | EPA DSSTox | |
URL | https://comptox.epa.gov/dashboard/DTXSID40942899 | |
Description | DSSTox provides a high quality public chemistry resource for supporting improved predictive toxicology. | |
Record name | 2-(3-Chloro-phenyl)-oxirane | |
Source | European Chemicals Agency (ECHA) | |
URL | https://echa.europa.eu/information-on-chemicals | |
Description | The European Chemicals Agency (ECHA) is an agency of the European Union which is the driving force among regulatory authorities in implementing the EU's groundbreaking chemicals legislation for the benefit of human health and the environment as well as for innovation and competitiveness. | |
Explanation | Use of the information, documents and data from the ECHA website is subject to the terms and conditions of this Legal Notice, and subject to other binding limitations provided for under applicable law, the information, documents and data made available on the ECHA website may be reproduced, distributed and/or used, totally or in part, for non-commercial purposes provided that ECHA is acknowledged as the source: "Source: European Chemicals Agency, http://echa.europa.eu/". Such acknowledgement must be included in each copy of the material. ECHA permits and encourages organisations and individuals to create links to the ECHA website under the following cumulative conditions: Links can only be made to webpages that provide a link to the Legal Notice page. | |
Synthesis routes and methods I
Procedure details
Synthesis routes and methods II
Procedure details
Synthesis routes and methods III
Procedure details
Retrosynthesis Analysis
AI-Powered Synthesis Planning: Our tool employs the Template_relevance Pistachio, Template_relevance Bkms_metabolic, Template_relevance Pistachio_ringbreaker, Template_relevance Reaxys, Template_relevance Reaxys_biocatalysis model, leveraging a vast database of chemical reactions to predict feasible synthetic routes.
One-Step Synthesis Focus: Specifically designed for one-step synthesis, it provides concise and direct routes for your target compounds, streamlining the synthesis process.
Accurate Predictions: Utilizing the extensive PISTACHIO, BKMS_METABOLIC, PISTACHIO_RINGBREAKER, REAXYS, REAXYS_BIOCATALYSIS database, our tool offers high-accuracy predictions, reflecting the latest in chemical research and data.
Strategy Settings
Precursor scoring | Relevance Heuristic |
---|---|
Min. plausibility | 0.01 |
Model | Template_relevance |
Template Set | Pistachio/Bkms_metabolic/Pistachio_ringbreaker/Reaxys/Reaxys_biocatalysis |
Top-N result to add to graph | 6 |
Feasible Synthetic Routes
Disclaimer and Information on In-Vitro Research Products
Please be aware that all articles and product information presented on BenchChem are intended solely for informational purposes. The products available for purchase on BenchChem are specifically designed for in-vitro studies, which are conducted outside of living organisms. In-vitro studies, derived from the Latin term "in glass," involve experiments performed in controlled laboratory settings using cells or tissues. It is important to note that these products are not categorized as medicines or drugs, and they have not received approval from the FDA for the prevention, treatment, or cure of any medical condition, ailment, or disease. We must emphasize that any form of bodily introduction of these products into humans or animals is strictly prohibited by law. It is essential to adhere to these guidelines to ensure compliance with legal and ethical standards in research and experimentation.