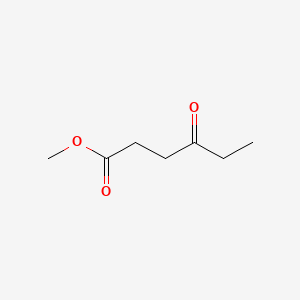
Methyl 4-oxohexanoate
Overview
Description
Preparation Methods
Synthetic Routes and Reaction Conditions: Methyl 4-oxohexanoate can be synthesized through several methods. One common method involves the reaction of butyraldehyde with methyl acrylate in the presence of a free radical initiator such as benzoyl peroxide. The reaction is carried out under reflux conditions with UV irradiation, leading to the formation of the desired product .
Industrial Production Methods: In industrial settings, this compound is often produced via the esterification of 4-oxohexanoic acid with methanol. This process typically involves the use of acid catalysts such as sulfuric acid or p-toluenesulfonic acid to facilitate the reaction. The reaction mixture is then purified through distillation to obtain the final product .
Chemical Reactions Analysis
Types of Reactions: Methyl 4-oxohexanoate undergoes various chemical reactions, including:
Oxidation: It can be oxidized to form 4-oxohexanoic acid.
Reduction: It can be reduced to form 4-hydroxyhexanoate.
Substitution: It can undergo nucleophilic substitution reactions to form various derivatives.
Common Reagents and Conditions:
Oxidation: Common oxidizing agents include potassium permanganate and chromium trioxide.
Reduction: Reducing agents such as sodium borohydride or lithium aluminum hydride are typically used.
Substitution: Nucleophiles such as amines or alcohols can be used under basic or acidic conditions.
Major Products Formed:
Oxidation: 4-oxohexanoic acid.
Reduction: 4-hydroxyhexanoate.
Substitution: Various substituted hexanoates depending on the nucleophile used.
Scientific Research Applications
Methyl 4-oxohexanoate has a wide range of applications in scientific research:
Chemistry: It is used as an intermediate in the synthesis of various organic compounds.
Biology: It serves as a precursor for the synthesis of biologically active molecules.
Medicine: It is used in the development of pharmaceuticals and drug delivery systems.
Industry: It is employed in the production of fragrances, flavors, and other specialty chemicals
Mechanism of Action
The mechanism of action of methyl 4-oxohexanoate involves its interaction with various molecular targets and pathways. For instance, in biological systems, it can undergo enzymatic transformations to form active metabolites that exert specific effects. The exact molecular targets and pathways depend on the specific application and context in which the compound is used .
Comparison with Similar Compounds
Ethyl 4-oxohexanoate: Similar structure but with an ethyl ester group instead of a methyl ester group.
Methyl 4-oxopentanoate: Similar structure but with one less carbon in the chain.
Methyl 4-oxoheptanoate: Similar structure but with one more carbon in the chain.
Uniqueness: Methyl 4-oxohexanoate is unique due to its specific chain length and functional groups, which confer distinct chemical and physical properties. These properties make it particularly useful in certain synthetic and industrial applications where other similar compounds may not be as effective .
Properties
IUPAC Name |
methyl 4-oxohexanoate | |
---|---|---|
Source | PubChem | |
URL | https://pubchem.ncbi.nlm.nih.gov | |
Description | Data deposited in or computed by PubChem | |
InChI |
InChI=1S/C7H12O3/c1-3-6(8)4-5-7(9)10-2/h3-5H2,1-2H3 | |
Source | PubChem | |
URL | https://pubchem.ncbi.nlm.nih.gov | |
Description | Data deposited in or computed by PubChem | |
InChI Key |
FOGHPENQGFYWSI-UHFFFAOYSA-N | |
Source | PubChem | |
URL | https://pubchem.ncbi.nlm.nih.gov | |
Description | Data deposited in or computed by PubChem | |
Canonical SMILES |
CCC(=O)CCC(=O)OC | |
Source | PubChem | |
URL | https://pubchem.ncbi.nlm.nih.gov | |
Description | Data deposited in or computed by PubChem | |
Molecular Formula |
C7H12O3 | |
Source | PubChem | |
URL | https://pubchem.ncbi.nlm.nih.gov | |
Description | Data deposited in or computed by PubChem | |
DSSTOX Substance ID |
DTXSID70338089 | |
Record name | Methyl 4-oxohexanoate | |
Source | EPA DSSTox | |
URL | https://comptox.epa.gov/dashboard/DTXSID70338089 | |
Description | DSSTox provides a high quality public chemistry resource for supporting improved predictive toxicology. | |
Molecular Weight |
144.17 g/mol | |
Source | PubChem | |
URL | https://pubchem.ncbi.nlm.nih.gov | |
Description | Data deposited in or computed by PubChem | |
CAS No. |
2955-62-6 | |
Record name | Methyl 4-oxohexanoate | |
Source | EPA DSSTox | |
URL | https://comptox.epa.gov/dashboard/DTXSID70338089 | |
Description | DSSTox provides a high quality public chemistry resource for supporting improved predictive toxicology. | |
Synthesis routes and methods I
Procedure details
Synthesis routes and methods II
Procedure details
Retrosynthesis Analysis
AI-Powered Synthesis Planning: Our tool employs the Template_relevance Pistachio, Template_relevance Bkms_metabolic, Template_relevance Pistachio_ringbreaker, Template_relevance Reaxys, Template_relevance Reaxys_biocatalysis model, leveraging a vast database of chemical reactions to predict feasible synthetic routes.
One-Step Synthesis Focus: Specifically designed for one-step synthesis, it provides concise and direct routes for your target compounds, streamlining the synthesis process.
Accurate Predictions: Utilizing the extensive PISTACHIO, BKMS_METABOLIC, PISTACHIO_RINGBREAKER, REAXYS, REAXYS_BIOCATALYSIS database, our tool offers high-accuracy predictions, reflecting the latest in chemical research and data.
Strategy Settings
Precursor scoring | Relevance Heuristic |
---|---|
Min. plausibility | 0.01 |
Model | Template_relevance |
Template Set | Pistachio/Bkms_metabolic/Pistachio_ringbreaker/Reaxys/Reaxys_biocatalysis |
Top-N result to add to graph | 6 |
Feasible Synthetic Routes
Q1: How is Methyl 4-oxohexanoate synthesized, and what unique regioselectivity is observed?
A1: this compound (8) is synthesized via a photochemical chlorocarbonylation reaction using 3-pentanone as the starting material []. The reaction involves irradiating a mixture of 3-pentanone and oxalyl chloride, followed by esterification with methanol. Notably, this reaction exhibits remarkable regioselectivity, favoring the formation of the γ-substituted product (this compound) over potential β-substituted isomers []. This regioselectivity is a key finding of the research and highlights the potential of photochemical chlorocarbonylation for controlled and selective synthesis.
Q2: Are there any applications of this compound derivatives in drug development?
A2: While not directly addressed in the provided research, a derivative of this compound, specifically 3-(9-acridinylamino)-5-hydroxymethylaniline N-(5-methyl-4-oxohexanoate), has been investigated for its potential in anticancer drug delivery []. This derivative serves as a linker between a water-soluble polymer carrier and an acridine-type anticancer drug. The hydrazone bond formed with the this compound moiety is designed to be hydrolytically labile and exhibits pH-controlled cleavage. This property allows for targeted drug release in the mildly acidic environment of tumor tissues [].
Q3: Can you elaborate on the significance of the hydrazone bond in the this compound derivative for drug delivery?
A3: The hydrazone bond within the 3-(9-acridinylamino)-5-hydroxymethylaniline N-(5-methyl-4-oxohexanoate) derivative plays a critical role in its potential as a drug delivery system. This bond exhibits pH-dependent stability, being relatively stable at physiological pH (7.4) but readily hydrolyzed in mildly acidic environments (pH 5.0), such as those found within tumor cells and late endosomes []. This characteristic allows for controlled drug release specifically within tumor tissues, potentially minimizing systemic toxicity and enhancing therapeutic efficacy. This approach highlights the importance of chemical modifications and linker design in developing targeted drug delivery strategies.
Disclaimer and Information on In-Vitro Research Products
Please be aware that all articles and product information presented on BenchChem are intended solely for informational purposes. The products available for purchase on BenchChem are specifically designed for in-vitro studies, which are conducted outside of living organisms. In-vitro studies, derived from the Latin term "in glass," involve experiments performed in controlled laboratory settings using cells or tissues. It is important to note that these products are not categorized as medicines or drugs, and they have not received approval from the FDA for the prevention, treatment, or cure of any medical condition, ailment, or disease. We must emphasize that any form of bodily introduction of these products into humans or animals is strictly prohibited by law. It is essential to adhere to these guidelines to ensure compliance with legal and ethical standards in research and experimentation.