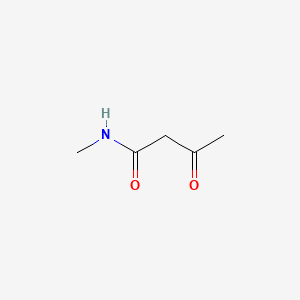
N-Methylacetoacetamide
Overview
Description
N-Methylacetoacetamide is a fatty amide . It has a molecular formula of C5H9NO2 and a molecular weight of 115.13 . It is a clear liquid that can range in color from light yellow to orange .
Synthesis Analysis
N-Methylacetoacetamide is a starting material in the synthesis of 1-Carbamoyl-2-oxopropyl acetate with the aid of (diacetoxyiodo)benzene (DIB) as the oxidant . It is also used as a reagent in the synthesis of 10H-Phenothiazines .Molecular Structure Analysis
The IUPAC Standard InChI for N-Methylacetoacetamide is InChI=1S/C3H7NO/c1-3(5)4-2/h1-2H3, (H,4,5) . The structure is available as a 2D Mol file or as a computed 3D SD file .Chemical Reactions Analysis
N-Methylacetoacetamide is a starting material in the synthesis of 1-Carbamoyl-2-oxopropyl acetate . It is also used as a reagent in the synthesis of 10H-Phenothiazines .Physical And Chemical Properties Analysis
N-Methylacetoacetamide has a melting point of -3 °C, a boiling point of 106°C, and a density of 1.069 . It has a refractive index of 1.4325-1.4345 . Its flash point is 105 °C and it should be stored at a temperature of 2-8°C . It is soluble in water through hydrolysis .Scientific Research Applications
Antidepressant Effects
N-Methylacetoacetamide, as a part of the N-methyl-D-aspartate (NMDA) receptor antagonists group, has been studied for its potential antidepressant effects. Research indicates that NMDA receptor-modulating drugs like N-Methylacetoacetamide can play a role in treating depression. For instance, Berman et al. (2000) found significant improvement in depressive symptoms in patients following treatment with NMDA receptor antagonists (Berman et al., 2000). Further, Krystal et al. (2013) discussed the rapid-acting antidepressant effects of such compounds, highlighting their potential in addressing major depressive episodes (Krystal et al., 2013).
Interaction with Ions in Aqueous Solutions
The interaction of N-Methylacetoacetamide with ions in aqueous solutions has been a subject of research. Pluhařová et al. (2014) studied how alkali cations and alkaline earth dications interact with the carbonyl group of aqueous N-Methylacetoacetamide, providing insights into its chemical behavior in different environments (Pluhařová et al., 2014).
Infrared Spectral Analysis
The infrared spectrum of N-Methylacetoacetamide, particularly the amide I, II, and III bands, is important for understanding its chemical properties. Ji et al. (2020) conducted a study to explain the formation of the amide infrared spectrum, contributing to a better understanding of organic chemistry and chemical biology (Yan Ji et al., 2020).
Hydrolysis Kinetics in High-Temperature Water
N-Methylacetamide's hydrolysis kinetics in high-temperature water has been explored to understand its behavior under extreme conditions. Duan et al. (2010) investigated the hydrolysis reaction of N-Methylacetamide in subcritical and supercritical water, which has implications for its stability and reactivity in industrial processes (Duan et al., 2010).
Molecular Structure Analysis
Studies on the molecular structure of N-Methylacetoacetamide provide insights into its physical and chemical properties. For instance, Kimura and Aoki (1953) investigated the molecular structures of N-Methylacetoacetamide using electron diffraction, offering valuable data for understanding its behavior in different states (Kimura & Aoki, 1953).
Safety and Hazards
N-Methylacetoacetamide is classified as harmful if swallowed, causes severe skin burns and eye damage, and may cause an allergic skin reaction . Precautionary measures include avoiding breathing dust, washing skin thoroughly after handling, not eating, drinking, or smoking when using this product, and wearing protective gloves, clothing, eye protection, and face protection .
Relevant Papers N-Methylacetoacetamide is a starting material in the synthesis of 1-Carbamoyl-2-oxopropyl acetate . It is also used as a reagent in the synthesis of 10H-Phenothiazines . These syntheses and their potential applications are discussed in various papers.
Mechanism of Action
Mode of Action
It is suggested that it may interact with its targets through hydrogen bonds , but more detailed studies are required to fully understand the compound’s interaction with its targets and any resulting changes .
Biochemical Pathways
The biochemical pathways affected by N-Methylacetoacetamide are not well-documented. It is possible that the compound could affect various pathways depending on its targets. Without specific information on its targets, it is difficult to summarize the affected pathways and their downstream effects .
Pharmacokinetics
Therefore, it is challenging to outline these properties and their impact on the compound’s bioavailability .
properties
IUPAC Name |
N-methyl-3-oxobutanamide | |
---|---|---|
Source | PubChem | |
URL | https://pubchem.ncbi.nlm.nih.gov | |
Description | Data deposited in or computed by PubChem | |
InChI |
InChI=1S/C5H9NO2/c1-4(7)3-5(8)6-2/h3H2,1-2H3,(H,6,8) | |
Source | PubChem | |
URL | https://pubchem.ncbi.nlm.nih.gov | |
Description | Data deposited in or computed by PubChem | |
InChI Key |
ATWLCPHWYPSRBQ-UHFFFAOYSA-N | |
Source | PubChem | |
URL | https://pubchem.ncbi.nlm.nih.gov | |
Description | Data deposited in or computed by PubChem | |
Canonical SMILES |
CC(=O)CC(=O)NC | |
Source | PubChem | |
URL | https://pubchem.ncbi.nlm.nih.gov | |
Description | Data deposited in or computed by PubChem | |
Molecular Formula |
C5H9NO2 | |
Source | PubChem | |
URL | https://pubchem.ncbi.nlm.nih.gov | |
Description | Data deposited in or computed by PubChem | |
DSSTOX Substance ID |
DTXSID9040138 | |
Record name | N-Methylacetoacetamide | |
Source | EPA DSSTox | |
URL | https://comptox.epa.gov/dashboard/DTXSID9040138 | |
Description | DSSTox provides a high quality public chemistry resource for supporting improved predictive toxicology. | |
Molecular Weight |
115.13 g/mol | |
Source | PubChem | |
URL | https://pubchem.ncbi.nlm.nih.gov | |
Description | Data deposited in or computed by PubChem | |
Product Name |
N-Methylacetoacetamide | |
CAS RN |
20306-75-6 | |
Record name | N-Methylacetoacetamide | |
Source | CAS Common Chemistry | |
URL | https://commonchemistry.cas.org/detail?cas_rn=20306-75-6 | |
Description | CAS Common Chemistry is an open community resource for accessing chemical information. Nearly 500,000 chemical substances from CAS REGISTRY cover areas of community interest, including common and frequently regulated chemicals, and those relevant to high school and undergraduate chemistry classes. This chemical information, curated by our expert scientists, is provided in alignment with our mission as a division of the American Chemical Society. | |
Explanation | The data from CAS Common Chemistry is provided under a CC-BY-NC 4.0 license, unless otherwise stated. | |
Record name | N-Methylacetoacetamide | |
Source | ChemIDplus | |
URL | https://pubchem.ncbi.nlm.nih.gov/substance/?source=chemidplus&sourceid=0020306756 | |
Description | ChemIDplus is a free, web search system that provides access to the structure and nomenclature authority files used for the identification of chemical substances cited in National Library of Medicine (NLM) databases, including the TOXNET system. | |
Record name | Butanamide, N-methyl-3-oxo- | |
Source | EPA Chemicals under the TSCA | |
URL | https://www.epa.gov/chemicals-under-tsca | |
Description | EPA Chemicals under the Toxic Substances Control Act (TSCA) collection contains information on chemicals and their regulations under TSCA, including non-confidential content from the TSCA Chemical Substance Inventory and Chemical Data Reporting. | |
Record name | N-Methylacetoacetamide | |
Source | EPA DSSTox | |
URL | https://comptox.epa.gov/dashboard/DTXSID9040138 | |
Description | DSSTox provides a high quality public chemistry resource for supporting improved predictive toxicology. | |
Record name | N-methyl-3-oxobutyramide | |
Source | European Chemicals Agency (ECHA) | |
URL | https://echa.europa.eu/substance-information/-/substanceinfo/100.039.733 | |
Description | The European Chemicals Agency (ECHA) is an agency of the European Union which is the driving force among regulatory authorities in implementing the EU's groundbreaking chemicals legislation for the benefit of human health and the environment as well as for innovation and competitiveness. | |
Explanation | Use of the information, documents and data from the ECHA website is subject to the terms and conditions of this Legal Notice, and subject to other binding limitations provided for under applicable law, the information, documents and data made available on the ECHA website may be reproduced, distributed and/or used, totally or in part, for non-commercial purposes provided that ECHA is acknowledged as the source: "Source: European Chemicals Agency, http://echa.europa.eu/". Such acknowledgement must be included in each copy of the material. ECHA permits and encourages organisations and individuals to create links to the ECHA website under the following cumulative conditions: Links can only be made to webpages that provide a link to the Legal Notice page. | |
Record name | N-METHYLACETOACETAMIDE | |
Source | FDA Global Substance Registration System (GSRS) | |
URL | https://gsrs.ncats.nih.gov/ginas/app/beta/substances/PWE9P6V839 | |
Description | The FDA Global Substance Registration System (GSRS) enables the efficient and accurate exchange of information on what substances are in regulated products. Instead of relying on names, which vary across regulatory domains, countries, and regions, the GSRS knowledge base makes it possible for substances to be defined by standardized, scientific descriptions. | |
Explanation | Unless otherwise noted, the contents of the FDA website (www.fda.gov), both text and graphics, are not copyrighted. They are in the public domain and may be republished, reprinted and otherwise used freely by anyone without the need to obtain permission from FDA. Credit to the U.S. Food and Drug Administration as the source is appreciated but not required. | |
Retrosynthesis Analysis
AI-Powered Synthesis Planning: Our tool employs the Template_relevance Pistachio, Template_relevance Bkms_metabolic, Template_relevance Pistachio_ringbreaker, Template_relevance Reaxys, Template_relevance Reaxys_biocatalysis model, leveraging a vast database of chemical reactions to predict feasible synthetic routes.
One-Step Synthesis Focus: Specifically designed for one-step synthesis, it provides concise and direct routes for your target compounds, streamlining the synthesis process.
Accurate Predictions: Utilizing the extensive PISTACHIO, BKMS_METABOLIC, PISTACHIO_RINGBREAKER, REAXYS, REAXYS_BIOCATALYSIS database, our tool offers high-accuracy predictions, reflecting the latest in chemical research and data.
Strategy Settings
Precursor scoring | Relevance Heuristic |
---|---|
Min. plausibility | 0.01 |
Model | Template_relevance |
Template Set | Pistachio/Bkms_metabolic/Pistachio_ringbreaker/Reaxys/Reaxys_biocatalysis |
Top-N result to add to graph | 6 |
Feasible Synthetic Routes
Disclaimer and Information on In-Vitro Research Products
Please be aware that all articles and product information presented on BenchChem are intended solely for informational purposes. The products available for purchase on BenchChem are specifically designed for in-vitro studies, which are conducted outside of living organisms. In-vitro studies, derived from the Latin term "in glass," involve experiments performed in controlled laboratory settings using cells or tissues. It is important to note that these products are not categorized as medicines or drugs, and they have not received approval from the FDA for the prevention, treatment, or cure of any medical condition, ailment, or disease. We must emphasize that any form of bodily introduction of these products into humans or animals is strictly prohibited by law. It is essential to adhere to these guidelines to ensure compliance with legal and ethical standards in research and experimentation.