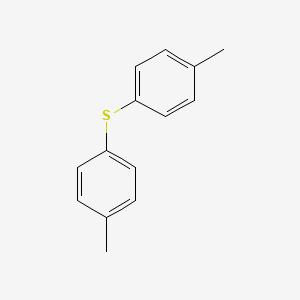
Di-p-tolyl sulphide
Overview
Description
Di-p-tolyl sulphide is an organic compound characterized by the presence of two p-tolyl groups attached to a sulphur atom. It is known for its applications in various fields due to its unique chemical properties. The compound is often used as a load-carrying additive in lubricants because of its anti-wear and extreme load-bearing qualities .
Mechanism of Action
Target of Action
Di-p-tolyl sulphide is primarily used as a load-carrying additive . Its main targets are the mechanical systems where it is applied, such as in lubricants and energy storage devices . It acts by reducing wear and increasing load-bearing qualities .
Biochemical Pathways
It’s known that the compound plays a significant role in energy storage devices . Upon lithiation, MoS2 expands up to 103%, which causes stress and affects battery stability and performance . This compound helps to manage this stress .
Pharmacokinetics
Its use in industrial applications suggests that it has good stability and can withstand various physical conditions .
Result of Action
The primary result of this compound’s action is the enhancement of anti-wear and extreme load-bearing qualities in the systems where it is applied . This leads to improved performance and longevity of these systems .
Action Environment
The action of this compound is influenced by environmental factors such as pressure and temperature . For instance, external pressure triggers a conformational up-conversion in the molecules of this compound . Additionally, the phase transitions in this compound occur at specific pressures and temperatures .
Biochemical Analysis
Biochemical Properties
It is known that disulfide bonds play a crucial role in the folding and structural stabilization of many important extracellular peptide and protein molecules, including hormones, enzymes, growth factors, toxins, and immunoglobulins . The formation of disulfide bonds in peptides and proteins is catalyzed by similar pathways in prokaryotes and eukaryotes .
Cellular Effects
It is known that disulfide bonds, such as those in Di-p-tolyl sulphide, can influence cell function
Molecular Mechanism
It is known that external pressure can trigger conformational changes in the molecules of this compound, leading to phase transition . This suggests that this compound may interact with biomolecules in a way that influences their conformation and function.
Temporal Effects in Laboratory Settings
In laboratory settings, the effects of this compound can change over time. For example, it has been observed that the phase transition between different forms of this compound can occur at different pressures and temperatures
Metabolic Pathways
It is known that disulfide bonds, such as those in this compound, can play a role in various metabolic processes .
Preparation Methods
Synthetic Routes and Reaction Conditions: Di-p-tolyl sulphide can be synthesized through several methods. One common method involves the reaction of p-tolyl magnesium bromide with sulphur dichloride. The reaction typically occurs in an inert atmosphere to prevent oxidation and is carried out at low temperatures to control the reaction rate.
Industrial Production Methods: In industrial settings, this compound is produced by the reaction of p-tolyl chloride with sodium sulphide. This method is preferred due to its cost-effectiveness and scalability. The reaction is usually conducted in a solvent such as ethanol or water, and the product is purified through distillation or recrystallization .
Chemical Reactions Analysis
Types of Reactions: Di-p-tolyl sulphide undergoes various chemical reactions, including:
Oxidation: It can be oxidized to form di-p-tolyl sulfoxide and di-p-tolyl sulfone.
Reduction: The compound can be reduced to form p-tolyl thiol.
Substitution: It can undergo substitution reactions where the p-tolyl groups are replaced by other functional groups.
Common Reagents and Conditions:
Oxidation: Common oxidizing agents include hydrogen peroxide and potassium permanganate.
Reduction: Reducing agents such as lithium aluminium hydride are used.
Substitution: Reagents like halogens or alkylating agents are employed under controlled conditions.
Major Products:
Oxidation: Di-p-tolyl sulfoxide and di-p-tolyl sulfone.
Reduction: p-Tolyl thiol.
Substitution: Various substituted derivatives depending on the reagents used.
Scientific Research Applications
Di-p-tolyl sulphide has a wide range of applications in scientific research:
Chemistry: It is used as a reagent in organic synthesis and as a stabilizer in polymer chemistry.
Biology: The compound is studied for its potential biological activities, including antimicrobial properties.
Medicine: Research is ongoing to explore its potential as a therapeutic agent due to its unique chemical structure.
Industry: It is widely used in the production of lubricants, pesticides, and energy storage devices.
Comparison with Similar Compounds
Di-p-tolyl sulphide can be compared with other similar compounds such as:
Di-p-tolyl disulfide: Similar in structure but contains an additional sulphur atom, leading to different chemical properties and applications.
Diphenyl sulphide: Contains phenyl groups instead of p-tolyl groups, resulting in different reactivity and applications.
Di-p-tolyl sulfoxide: An oxidized form of this compound with different chemical properties and uses.
Uniqueness: this compound is unique due to its specific structure, which imparts distinct chemical properties such as high thermal stability and resistance to oxidation. These properties make it particularly useful in applications requiring high-performance materials .
Properties
IUPAC Name |
1-methyl-4-(4-methylphenyl)sulfanylbenzene | |
---|---|---|
Source | PubChem | |
URL | https://pubchem.ncbi.nlm.nih.gov | |
Description | Data deposited in or computed by PubChem | |
InChI |
InChI=1S/C14H14S/c1-11-3-7-13(8-4-11)15-14-9-5-12(2)6-10-14/h3-10H,1-2H3 | |
Source | PubChem | |
URL | https://pubchem.ncbi.nlm.nih.gov | |
Description | Data deposited in or computed by PubChem | |
InChI Key |
NRXWFTYEJYEOGW-UHFFFAOYSA-N | |
Source | PubChem | |
URL | https://pubchem.ncbi.nlm.nih.gov | |
Description | Data deposited in or computed by PubChem | |
Canonical SMILES |
CC1=CC=C(C=C1)SC2=CC=C(C=C2)C | |
Source | PubChem | |
URL | https://pubchem.ncbi.nlm.nih.gov | |
Description | Data deposited in or computed by PubChem | |
Molecular Formula |
C14H14S | |
Source | PubChem | |
URL | https://pubchem.ncbi.nlm.nih.gov | |
Description | Data deposited in or computed by PubChem | |
DSSTOX Substance ID |
DTXSID20211050 | |
Record name | Di-p-tolyl sulphide | |
Source | EPA DSSTox | |
URL | https://comptox.epa.gov/dashboard/DTXSID20211050 | |
Description | DSSTox provides a high quality public chemistry resource for supporting improved predictive toxicology. | |
Molecular Weight |
214.33 g/mol | |
Source | PubChem | |
URL | https://pubchem.ncbi.nlm.nih.gov | |
Description | Data deposited in or computed by PubChem | |
CAS No. |
620-94-0 | |
Record name | 1,1′-Thiobis[4-methylbenzene] | |
Source | CAS Common Chemistry | |
URL | https://commonchemistry.cas.org/detail?cas_rn=620-94-0 | |
Description | CAS Common Chemistry is an open community resource for accessing chemical information. Nearly 500,000 chemical substances from CAS REGISTRY cover areas of community interest, including common and frequently regulated chemicals, and those relevant to high school and undergraduate chemistry classes. This chemical information, curated by our expert scientists, is provided in alignment with our mission as a division of the American Chemical Society. | |
Explanation | The data from CAS Common Chemistry is provided under a CC-BY-NC 4.0 license, unless otherwise stated. | |
Record name | Di-p-tolyl sulphide | |
Source | ChemIDplus | |
URL | https://pubchem.ncbi.nlm.nih.gov/substance/?source=chemidplus&sourceid=0000620940 | |
Description | ChemIDplus is a free, web search system that provides access to the structure and nomenclature authority files used for the identification of chemical substances cited in National Library of Medicine (NLM) databases, including the TOXNET system. | |
Record name | Di-p-tolyl sulphide | |
Source | EPA DSSTox | |
URL | https://comptox.epa.gov/dashboard/DTXSID20211050 | |
Description | DSSTox provides a high quality public chemistry resource for supporting improved predictive toxicology. | |
Record name | Di-p-tolyl sulphide | |
Source | European Chemicals Agency (ECHA) | |
URL | https://echa.europa.eu/substance-information/-/substanceinfo/100.009.693 | |
Description | The European Chemicals Agency (ECHA) is an agency of the European Union which is the driving force among regulatory authorities in implementing the EU's groundbreaking chemicals legislation for the benefit of human health and the environment as well as for innovation and competitiveness. | |
Explanation | Use of the information, documents and data from the ECHA website is subject to the terms and conditions of this Legal Notice, and subject to other binding limitations provided for under applicable law, the information, documents and data made available on the ECHA website may be reproduced, distributed and/or used, totally or in part, for non-commercial purposes provided that ECHA is acknowledged as the source: "Source: European Chemicals Agency, http://echa.europa.eu/". Such acknowledgement must be included in each copy of the material. ECHA permits and encourages organisations and individuals to create links to the ECHA website under the following cumulative conditions: Links can only be made to webpages that provide a link to the Legal Notice page. | |
Retrosynthesis Analysis
AI-Powered Synthesis Planning: Our tool employs the Template_relevance Pistachio, Template_relevance Bkms_metabolic, Template_relevance Pistachio_ringbreaker, Template_relevance Reaxys, Template_relevance Reaxys_biocatalysis model, leveraging a vast database of chemical reactions to predict feasible synthetic routes.
One-Step Synthesis Focus: Specifically designed for one-step synthesis, it provides concise and direct routes for your target compounds, streamlining the synthesis process.
Accurate Predictions: Utilizing the extensive PISTACHIO, BKMS_METABOLIC, PISTACHIO_RINGBREAKER, REAXYS, REAXYS_BIOCATALYSIS database, our tool offers high-accuracy predictions, reflecting the latest in chemical research and data.
Strategy Settings
Precursor scoring | Relevance Heuristic |
---|---|
Min. plausibility | 0.01 |
Model | Template_relevance |
Template Set | Pistachio/Bkms_metabolic/Pistachio_ringbreaker/Reaxys/Reaxys_biocatalysis |
Top-N result to add to graph | 6 |
Feasible Synthetic Routes
Disclaimer and Information on In-Vitro Research Products
Please be aware that all articles and product information presented on BenchChem are intended solely for informational purposes. The products available for purchase on BenchChem are specifically designed for in-vitro studies, which are conducted outside of living organisms. In-vitro studies, derived from the Latin term "in glass," involve experiments performed in controlled laboratory settings using cells or tissues. It is important to note that these products are not categorized as medicines or drugs, and they have not received approval from the FDA for the prevention, treatment, or cure of any medical condition, ailment, or disease. We must emphasize that any form of bodily introduction of these products into humans or animals is strictly prohibited by law. It is essential to adhere to these guidelines to ensure compliance with legal and ethical standards in research and experimentation.