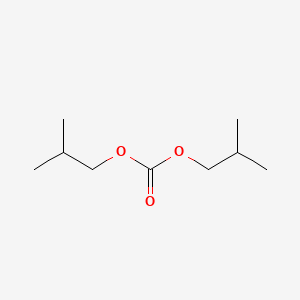
Diisobutyl carbonate
Overview
Description
Diisobutyl carbonate (CAS RN: 539-92-4) is a carbonate ester with the molecular formula C₉H₁₈O₃ and a molecular weight of 174.24 g/mol . Its IUPAC name is bis(2-methylpropyl) carbonate, and it is also known as carbonic acid, diisobutyl ester . The compound is characterized by two isobutyl groups attached to a central carbonate group.
Preparation Methods
Phosgene Method
The phosgene method involves the reaction of phosgene (COCl₂) with alcohols to form dialkyl carbonates. Although this method has been historically common, it has significant drawbacks:
- Toxicity : Phosgene is highly toxic and hazardous to handle.
- Equipment Corrosion : The process generates corrosive by-products.
- Environmental Concerns : The use of phosgene and its derivatives poses serious environmental risks.
- Complex Operation : Requires long reaction times and specialized equipment.
Due to these disadvantages, the phosgene method is being phased out in favor of safer alternatives.
Urea Alcoholysis Method
This method synthesizes dialkyl carbonates by reacting urea with alcohols. The process typically involves two steps:
- Formation of alkyl carbamate from urea and alcohol.
- Conversion of carbamate to dialkyl carbonate at high temperatures.
- The resonance stabilization of the amide group in urea makes the substitution by alkoxy groups thermodynamically difficult.
- Requires harsh reaction conditions (high temperature and pressure).
- Low reaction efficiency and yield.
- Complex two-step process increases equipment and operational costs.
- Large amounts of alcohol are needed, complicating product separation.
An improved variant involves using dimethyl carbonate and urea to first synthesize butyl carbamate, then converting it to dibutyl carbonate, but this still suffers from complexity and inefficiency.
Transesterification Method
The transesterification method is currently the most promising and environmentally friendly approach for diisobutyl carbonate synthesis. It involves the direct reaction of dimethyl carbonate with isobutanol under catalytic conditions to produce this compound and methanol as a by-product.
- Uses dimethyl carbonate, which is less toxic and more environmentally benign.
- Methanol by-product can be recycled to synthesize dimethyl carbonate, enhancing sustainability.
- Direct one-step synthesis simplifies the process.
- Good industrial scalability and economic feasibility.
Catalytic Synthesis Using Proline Ionic Liquid
A notable advancement in the transesterification method is the use of proline-based ionic liquid catalysts . This method was detailed in Chinese patent CN103709032B, which describes a catalytic synthesis of dibutyl carbonate (a close analog to this compound) using proline ionic liquids.
Process Summary:
Step | Description |
---|---|
1 | Replace air in the reactor with inert gas to create an inert atmosphere. Add dimethyl carbonate, isobutanol, and proline ionic liquid catalyst. Stir to mix evenly. |
2 | Heat the reactor to the target reaction temperature and maintain for a set reaction time. After completion, cool and separate phases. |
3 | The upper phase undergoes vacuum distillation at 100-105 °C to collect the colorless this compound product. The lower phase contains the ionic liquid catalyst and methanol by-product. |
4 | Methanol is recovered and recycled for dimethyl carbonate synthesis. The ionic liquid catalyst is viscous and can be reused after distillation. |
- The ionic liquid catalyst enhances reaction efficiency and selectivity.
- The process allows catalyst recycling, reducing costs and waste.
- Methanol recovery improves overall process sustainability.
- Operates under relatively mild conditions compared to urea alcoholysis.
Comparative Analysis of Preparation Methods
Method | Raw Materials | Catalyst | Reaction Conditions | By-products | Advantages | Disadvantages |
---|---|---|---|---|---|---|
Phosgene Method | Phosgene + Isobutanol | None or acid catalysts | Toxic, corrosive environment | HCl, other corrosives | High purity product | Toxicity, corrosion, environmental hazards |
Urea Alcoholysis Method | Urea + Isobutanol | None | High temperature and pressure | Ammonia, complex mixture | Avoids phosgene | Low efficiency, complex process |
Transesterification | Dimethyl carbonate + Isobutanol | Acid/base or ionic liquid catalysts | Moderate temperature, inert atmosphere | Methanol (recyclable) | Environmentally friendly, efficient, scalable | Requires catalyst optimization |
Proline Ionic Liquid Catalysis (Transesterification variant) | Dimethyl carbonate + Isobutanol | Proline-based ionic liquid | Mild to moderate temperature | Methanol (recyclable) | High selectivity, catalyst recycling, green process | Requires ionic liquid synthesis |
Research Findings and Industrial Implications
- The proline ionic liquid catalyzed transesterification method has been shown to achieve high yields of dialkyl carbonates with excellent selectivity and catalyst recyclability.
- Methanol recovery and recycling significantly reduce raw material costs and environmental impact.
- The process is adaptable to various alcohols, including isobutanol, facilitating the production of this compound.
- Industrial adoption of ionic liquid catalysis is promising due to reduced hazardous waste and improved process economics.
- Optimization of reaction parameters such as temperature, catalyst loading, and reaction time is crucial for maximizing yield and minimizing by-products.
Summary Table of Preparation Methods and Key Parameters
Parameter | Phosgene Method | Urea Alcoholysis Method | Transesterification (Conventional) | Proline Ionic Liquid Catalysis |
---|---|---|---|---|
Reaction Temperature (°C) | 50-100 | 150-200 | 80-120 | 80-110 |
Reaction Time (hours) | Several | Several | 4-8 | 3-6 |
Catalyst | Acid catalysts | None | Alkali or acid catalysts | Proline ionic liquid |
By-products | HCl, corrosive waste | Ammonia, urea residues | Methanol (recyclable) | Methanol (recyclable) |
Environmental Impact | High | Moderate | Low | Very low |
Catalyst Recyclability | No | No | Limited | Yes |
Industrial Feasibility | Declining | Limited | High | Emerging |
Chemical Reactions Analysis
Types of Reactions: Diisobutyl carbonate undergoes various chemical reactions, including:
Hydrolysis: Reacts with water to form isobutanol and carbon dioxide.
Transesterification: Reacts with alcohols to form different carbonate esters.
Reduction: Can be reduced to isobutanol using reducing agents.
Common Reagents and Conditions:
Hydrolysis: Water, acidic or basic conditions.
Transesterification: Alcohols, acidic or basic catalysts.
Reduction: Reducing agents such as lithium aluminum hydride.
Major Products Formed:
Hydrolysis: Isobutanol and carbon dioxide.
Transesterification: Different carbonate esters.
Reduction: Isobutanol.
Scientific Research Applications
Chemical Applications
1.1 Solvent and Intermediate in Organic Synthesis
DIBC is primarily utilized as a solvent in organic synthesis due to its excellent solvating properties for a variety of organic compounds. It facilitates chemical reactions by dissolving reactants and enhancing their reactivity. DIBC is also used as an intermediate in the production of other chemicals, particularly in the synthesis of dialkyl carbonates through transesterification processes.
1.2 Reactivity in Chemical Processes
DIBC participates in various chemical reactions, including hydrolysis and transesterification, leading to the formation of different products. Its ability to act as both a solvent and reactant makes it valuable in synthetic organic chemistry.
Biological Applications
2.1 Sample Preparation for Biological Analysis
In biological research, DIBC is employed for preparing biological samples for analysis. Its solvent properties allow it to dissolve biomolecules effectively, facilitating their study in various assays and experiments.
2.2 Drug Delivery Systems
Recent studies have investigated the potential of DIBC in drug delivery systems. Its low toxicity and favorable solvation characteristics make it a candidate for formulating drug carriers that can enhance the bioavailability of therapeutic agents.
Industrial Applications
3.1 Coatings and Adhesives
DIBC is used in the formulation of coatings and adhesives due to its ability to improve the physical properties of these materials. It enhances adhesion, flexibility, and durability, making it suitable for various industrial applications.
3.2 Plasticizers
In the plastics industry, DIBC serves as a plasticizer that improves the flexibility and workability of polymer materials. Its incorporation into plastic formulations helps achieve desired mechanical properties while maintaining product stability.
Synthesis and Use in Green Chemistry
A study conducted by researchers at The University of Queensland highlighted the use of DIBC in green chemical synthesis. The research focused on the production of dimethyl carbonate via carbonyl bromide intermediates, showcasing DIBC's role as a sustainable solvent alternative .
Valorization of Glycerol
Another significant application was reported in a study that explored the transesterification reaction of glycerol with dialkyl carbonates, including DIBC. This process demonstrated DIBC's potential as a reagent that can convert waste glycerol into valuable chemicals like glycerol carbonate and glycidol .
Mechanism of Action
Diisobutyl carbonate exerts its effects primarily through its ability to act as a solvent and reactant in various chemical processes. Its mechanism of action involves:
Solvent Properties: Dissolves a wide range of organic compounds, facilitating chemical reactions.
Reactivity: Participates in reactions such as hydrolysis and transesterification, leading to the formation of different products.
Comparison with Similar Compounds
Key Properties:
- Boiling Point: Not explicitly reported in available literature.
- Applications : Primarily used in industrial and scientific research , including synthesis of antifungal agents (e.g., phenylpyrrole-substituted tetramic acids) .
- Regulatory Status : Listed in the European Inventory of Existing Commercial Chemical Substances (EINECS) .
Diphenyl Carbonate (CAS RN: 102-09-0)
Molecular Formula : C₁₃H₁₀O₃
Molecular Weight : 214.21 g/mol .
- Applications: Widely used in polycarbonate production (e.g., plastics, optical media) due to its reactivity with bisphenol A .
- Key Differences :
Diisobutyl Adipate (No CAS Provided)
Molecular Formula : C₁₄H₂₆O₄ (inferred from adipic acid structure).
Molecular Weight : ~274.35 g/mol.
- Applications : Used as a plasticizer in polymers and emollient in cosmetics .
- Market Data : Global market valued at $12 million (2020) , projected to reach $16 million by 2026 (CAGR: 4.4%) .
- Key Differences :
Diisobutyl Glutarate and Succinate
Diisobutyl Glutarate :
Diisobutyl Succinate :
- Toxicology : Both compounds are data-poor , requiring read-across from dimethyl esters (e.g., dimethyl glutarate EU-LCI: 50 µg/m³) for risk assessment .
- Key Differences: Glutarate and succinate esters derive from dicarboxylic acids, whereas this compound is a monocarboxylic acid ester. this compound’s lower molecular weight may enhance volatility and reactivity in synthesis .
Diisobutyl Phthalate (DIBP; CAS RN: 84-69-5)
Molecular Formula : C₁₆H₂₂O₄.
Molecular Weight : 278.34 g/mol.
- Applications: Historically used as a plasticizer, now restricted due to toxicity concerns .
- Regulatory Status : Listed for ban/elimination under international chemical safety frameworks .
- Key Differences: DIBP is a phthalate ester linked to reproductive toxicity, unlike carbonate esters.
Comparative Data Table
Research Findings and Key Insights
- Synthetic Utility : this compound is critical in synthesizing antifungal agents (e.g., compound 6q, 76.7% yield) .
- Toxicology : Unlike diisobutyl phthalate, this compound lacks evidence of reproductive toxicity, though full toxicological profiles are incomplete .
- Market Trends : Adipate esters dominate industrial markets, while this compound remains a research-focused compound .
Biological Activity
Diisobutyl carbonate (DIBC), a carbonate ester with the chemical formula CHO, is primarily utilized as a solvent and in the synthesis of various organic compounds. Its biological activity has garnered attention in recent years, particularly regarding its potential applications in pharmaceuticals and toxicology.
Antimicrobial Properties
Recent studies have highlighted the antimicrobial activity of this compound. It has been evaluated against various bacterial strains, demonstrating effectiveness similar to that of established antimicrobial agents. For instance, a study assessed its inhibitory effects on Gram-positive and Gram-negative bacteria, revealing that DIBC exhibits significant antibacterial properties. The results indicated a minimum inhibitory concentration (MIC) that suggests its potential as an alternative antimicrobial agent in clinical settings.
Cytotoxicity and Antitumor Activity
Research has also explored the cytotoxic effects of this compound on cancer cell lines. In vitro studies have shown that DIBC can induce apoptosis in various tumor cell lines, including those derived from lung and breast cancers. The cytotoxicity was quantified using IC values, which indicate the concentration required to inhibit cell growth by 50%.
Cell Line | IC (μg/mL) | Time (h) |
---|---|---|
KYSE70 | 1.463 | 24 |
KYSE150 | 0.888 | 24 |
MCF-7 (Breast) | 2.5 | 48 |
These findings suggest that DIBC may possess anticancer properties , warranting further investigation into its mechanisms of action.
The mechanisms through which this compound exerts its biological effects are still under investigation. Preliminary studies indicate that it may interact with cellular pathways involved in apoptosis and cell cycle regulation. For example, DIBC has been shown to upregulate p21 expression, leading to cell cycle arrest in the G2/M phase, which is critical for cancer therapy strategies.
Case Study 1: Antimicrobial Efficacy
In a controlled laboratory setting, this compound was tested against clinical isolates of Staphylococcus aureus and Escherichia coli. The study utilized a disc diffusion method combined with serial dilution techniques to determine the effectiveness of DIBC as an antimicrobial agent.
Results:
- Staphylococcus aureus: MIC = 32 µg/mL
- Escherichia coli: MIC = 64 µg/mL
These results underscore the potential for DIBC to be developed as a novel antimicrobial treatment.
Case Study 2: Antitumor Activity
A study investigated the antitumor effects of this compound on human lung cancer cells (A549). The compound was administered at varying concentrations, and cell viability was assessed using an MTT assay.
Findings:
- At a concentration of 5 µg/mL, DIBC reduced cell viability by approximately 60% after 48 hours.
- Flow cytometric analysis indicated an increase in apoptotic cells in treated groups compared to controls.
These findings point to the promising role of this compound in cancer therapeutics.
Q & A
Basic Research Questions
Q. What are the key physicochemical properties of diisobutyl carbonate, and how do they influence experimental design?
this compound (CAS 539-92-4) has a molecular formula of C₉H₁₈O₃ and a molecular weight of 174.24 g/mol . Its density (~1.0475 g/mL) and boiling point (128.7°C) are critical for solvent selection and reaction optimization, especially in reflux conditions . The compound’s stability under standard storage conditions and incompatibility with oxidizing agents or bases necessitate inert atmospheres and dry environments during synthesis . For analytical workflows, its refractive index (1.4060–1.4080) can aid in purity assessment via refractometry .
Q. How can researchers safely handle and store this compound in laboratory settings?
Safe handling requires chemical-resistant gloves (JIS T 8116), protective eyewear (JIS T 8147), and ventilation to avoid inhalation or skin contact . Storage must prioritize airtight glass containers to prevent moisture ingress or decomposition. Electrostatic charge buildup should be mitigated during transfer . Stability data indicate that decomposition under heat produces carbon oxides, necessitating avoidance of open flames .
Q. What spectroscopic methods are recommended for characterizing this compound and its derivatives?
Infrared (IR) spectroscopy is essential for identifying carbonyl (C=O) and ester (C-O) functional groups, with authentic IR spectra serving as benchmarks . For structural elucidation, ¹³C NMR can resolve methyl, methylene, and quaternary carbons, as demonstrated for structurally similar diisobutyl phthalate (DEPT spectrum analysis in Table 2) . GC-MS is critical for detecting trace impurities like residual isobutyl chloroformate (<1.0%) during synthesis .
Advanced Research Questions
Q. How can read-across toxicological data address gaps in this compound’s safety profile?
this compound shares metabolic pathways with esters like dimethyl succinate, where carboxylesterase-mediated hydrolysis generates carboxylic acids and alcohols . Toxicological read-across from dimethyl glutarate (EU-LCI value: 50 µg/m³) is viable if structural and metabolic similarities are validated . However, in vitro assays (e.g., nasal mucosa models) are required to confirm enzymatic conversion rates and dose-response relationships .
Q. What synthetic strategies optimize the yield of this compound derivatives for antifungal applications?
Modifying the carbonate moiety with aromatic substituents (e.g., trifluoromethylphenyl groups) enhances bioactivity, as seen in pyrrole-substituted tetramic acid derivatives . Reaction conditions (e.g., 76.7% yield for 6q) highlight the importance of stoichiometric control and inert atmospheres to prevent side reactions. Post-synthesis purification via column chromatography (monitored by TLC) ensures structural fidelity .
Q. How do high-pressure conditions affect the thermal conductivity of diisobutyl sebacate analogs, and what implications does this have for material science?
Experimental studies on diisobutyl sebacate under high temperatures (1409–1411 K) and pressures reveal nonlinear thermal conductivity trends, attributed to molecular packing and intermolecular interactions . These findings suggest this compound derivatives could serve as heat-transfer fluids in extreme environments, provided phase stability is confirmed via differential scanning calorimetry (DSC) .
Q. What computational methods validate the use of this compound in green chemistry applications?
Molecular dynamics simulations can predict solvation effects in CO₂ capture or catalytic systems, leveraging its low viscosity and polarity. Pairing computational data with experimental metrics (e.g., Hansen solubility parameters) enables solvent selection for sustainable reaction media. However, empirical validation of biodegradability (e.g., OECD 301D assays) remains critical to avoid ecological risks .
Q. Methodological Notes
- Data Contradictions : While this compound’s stability is well-documented , conflicting reports on its reactivity with acids (e.g., potential decarboxylation) require validation via controlled kinetic studies.
- Analytical Pitfalls : GC assays may underestimate impurities if detector sensitivity is not calibrated for low-volatility byproducts .
- Toxicology Gaps : Absence of acute toxicity data necessitates precautionary exposure limits (e.g., adopting dimethyl glutarate’s EU-LCI until in vivo data are available) .
Properties
IUPAC Name |
bis(2-methylpropyl) carbonate | |
---|---|---|
Source | PubChem | |
URL | https://pubchem.ncbi.nlm.nih.gov | |
Description | Data deposited in or computed by PubChem | |
InChI |
InChI=1S/C9H18O3/c1-7(2)5-11-9(10)12-6-8(3)4/h7-8H,5-6H2,1-4H3 | |
Source | PubChem | |
URL | https://pubchem.ncbi.nlm.nih.gov | |
Description | Data deposited in or computed by PubChem | |
InChI Key |
UXXXZMDJQLPQPH-UHFFFAOYSA-N | |
Source | PubChem | |
URL | https://pubchem.ncbi.nlm.nih.gov | |
Description | Data deposited in or computed by PubChem | |
Canonical SMILES |
CC(C)COC(=O)OCC(C)C | |
Source | PubChem | |
URL | https://pubchem.ncbi.nlm.nih.gov | |
Description | Data deposited in or computed by PubChem | |
Molecular Formula |
C9H18O3 | |
Source | PubChem | |
URL | https://pubchem.ncbi.nlm.nih.gov | |
Description | Data deposited in or computed by PubChem | |
DSSTOX Substance ID |
DTXSID4060236 | |
Record name | Carbonic acid, bis(2-methylpropyl) ester | |
Source | EPA DSSTox | |
URL | https://comptox.epa.gov/dashboard/DTXSID4060236 | |
Description | DSSTox provides a high quality public chemistry resource for supporting improved predictive toxicology. | |
Molecular Weight |
174.24 g/mol | |
Source | PubChem | |
URL | https://pubchem.ncbi.nlm.nih.gov | |
Description | Data deposited in or computed by PubChem | |
CAS No. |
539-92-4 | |
Record name | Diisobutyl carbonate | |
Source | CAS Common Chemistry | |
URL | https://commonchemistry.cas.org/detail?cas_rn=539-92-4 | |
Description | CAS Common Chemistry is an open community resource for accessing chemical information. Nearly 500,000 chemical substances from CAS REGISTRY cover areas of community interest, including common and frequently regulated chemicals, and those relevant to high school and undergraduate chemistry classes. This chemical information, curated by our expert scientists, is provided in alignment with our mission as a division of the American Chemical Society. | |
Explanation | The data from CAS Common Chemistry is provided under a CC-BY-NC 4.0 license, unless otherwise stated. | |
Record name | Carbonic acid, bis(2-methylpropyl) ester | |
Source | ChemIDplus | |
URL | https://pubchem.ncbi.nlm.nih.gov/substance/?source=chemidplus&sourceid=0000539924 | |
Description | ChemIDplus is a free, web search system that provides access to the structure and nomenclature authority files used for the identification of chemical substances cited in National Library of Medicine (NLM) databases, including the TOXNET system. | |
Record name | Diisobutyl carbonate | |
Source | DTP/NCI | |
URL | https://dtp.cancer.gov/dtpstandard/servlet/dwindex?searchtype=NSC&outputformat=html&searchlist=4009 | |
Description | The NCI Development Therapeutics Program (DTP) provides services and resources to the academic and private-sector research communities worldwide to facilitate the discovery and development of new cancer therapeutic agents. | |
Explanation | Unless otherwise indicated, all text within NCI products is free of copyright and may be reused without our permission. Credit the National Cancer Institute as the source. | |
Record name | Carbonic acid, bis(2-methylpropyl) ester | |
Source | EPA Chemicals under the TSCA | |
URL | https://www.epa.gov/chemicals-under-tsca | |
Description | EPA Chemicals under the Toxic Substances Control Act (TSCA) collection contains information on chemicals and their regulations under TSCA, including non-confidential content from the TSCA Chemical Substance Inventory and Chemical Data Reporting. | |
Record name | Carbonic acid, bis(2-methylpropyl) ester | |
Source | EPA DSSTox | |
URL | https://comptox.epa.gov/dashboard/DTXSID4060236 | |
Description | DSSTox provides a high quality public chemistry resource for supporting improved predictive toxicology. | |
Record name | Diisobutyl carbonate | |
Source | European Chemicals Agency (ECHA) | |
URL | https://echa.europa.eu/substance-information/-/substanceinfo/100.007.938 | |
Description | The European Chemicals Agency (ECHA) is an agency of the European Union which is the driving force among regulatory authorities in implementing the EU's groundbreaking chemicals legislation for the benefit of human health and the environment as well as for innovation and competitiveness. | |
Explanation | Use of the information, documents and data from the ECHA website is subject to the terms and conditions of this Legal Notice, and subject to other binding limitations provided for under applicable law, the information, documents and data made available on the ECHA website may be reproduced, distributed and/or used, totally or in part, for non-commercial purposes provided that ECHA is acknowledged as the source: "Source: European Chemicals Agency, http://echa.europa.eu/". Such acknowledgement must be included in each copy of the material. ECHA permits and encourages organisations and individuals to create links to the ECHA website under the following cumulative conditions: Links can only be made to webpages that provide a link to the Legal Notice page. | |
Record name | CARBONIC ACID, BIS(2-METHYLPROPYL) ESTER | |
Source | FDA Global Substance Registration System (GSRS) | |
URL | https://gsrs.ncats.nih.gov/ginas/app/beta/substances/UN7NR8B1XJ | |
Description | The FDA Global Substance Registration System (GSRS) enables the efficient and accurate exchange of information on what substances are in regulated products. Instead of relying on names, which vary across regulatory domains, countries, and regions, the GSRS knowledge base makes it possible for substances to be defined by standardized, scientific descriptions. | |
Explanation | Unless otherwise noted, the contents of the FDA website (www.fda.gov), both text and graphics, are not copyrighted. They are in the public domain and may be republished, reprinted and otherwise used freely by anyone without the need to obtain permission from FDA. Credit to the U.S. Food and Drug Administration as the source is appreciated but not required. | |
Retrosynthesis Analysis
AI-Powered Synthesis Planning: Our tool employs the Template_relevance Pistachio, Template_relevance Bkms_metabolic, Template_relevance Pistachio_ringbreaker, Template_relevance Reaxys, Template_relevance Reaxys_biocatalysis model, leveraging a vast database of chemical reactions to predict feasible synthetic routes.
One-Step Synthesis Focus: Specifically designed for one-step synthesis, it provides concise and direct routes for your target compounds, streamlining the synthesis process.
Accurate Predictions: Utilizing the extensive PISTACHIO, BKMS_METABOLIC, PISTACHIO_RINGBREAKER, REAXYS, REAXYS_BIOCATALYSIS database, our tool offers high-accuracy predictions, reflecting the latest in chemical research and data.
Strategy Settings
Precursor scoring | Relevance Heuristic |
---|---|
Min. plausibility | 0.01 |
Model | Template_relevance |
Template Set | Pistachio/Bkms_metabolic/Pistachio_ringbreaker/Reaxys/Reaxys_biocatalysis |
Top-N result to add to graph | 6 |
Feasible Synthetic Routes
Disclaimer and Information on In-Vitro Research Products
Please be aware that all articles and product information presented on BenchChem are intended solely for informational purposes. The products available for purchase on BenchChem are specifically designed for in-vitro studies, which are conducted outside of living organisms. In-vitro studies, derived from the Latin term "in glass," involve experiments performed in controlled laboratory settings using cells or tissues. It is important to note that these products are not categorized as medicines or drugs, and they have not received approval from the FDA for the prevention, treatment, or cure of any medical condition, ailment, or disease. We must emphasize that any form of bodily introduction of these products into humans or animals is strictly prohibited by law. It is essential to adhere to these guidelines to ensure compliance with legal and ethical standards in research and experimentation.