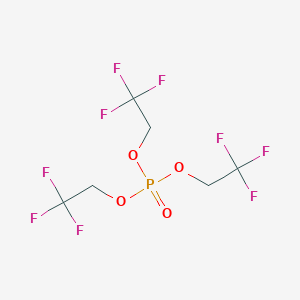
Tris(2,2,2-trifluoroethyl) phosphate
Overview
Description
Tris(2,2,2-trifluoroethyl) phosphate: is an organophosphorus compound with the molecular formula C6H6F9O4P. It is known for its unique properties, including high thermal stability and non-flammability, making it a valuable compound in various industrial applications .
Mechanism of Action
Target of Action
Tris(2,2,2-trifluoroethyl) phosphate primarily targets the electrolyte systems in rechargeable alkali metal ion batteries, such as lithium-ion batteries . It acts as a ligand in organometallic chemistry to enhance labialization in metal clusters .
Mode of Action
this compound interacts with its targets by acting as a substitutional equivalent for carbon monoxide . It also serves as an electrolyte additive to improve the electrochemical performance and as a co-solvent for nonflammable electrolytes in lithium-ion batteries .
Biochemical Pathways
The compound affects the biochemical pathways involved in the operation of rechargeable alkali metal ion batteries. It plays a crucial role in the formation of solid electrolyte interface (SEI) film on the graphite electrode . This interaction enhances the stability and performance of the batteries .
Pharmacokinetics
It improves the electrochemical performance of the batteries, indicating its effective distribution and interaction within the battery system .
Result of Action
The primary result of this compound’s action is the enhancement of the safety and performance of rechargeable alkali metal ion batteries. It contributes to the formation of a stable SEI film on the graphite electrode, which increases the thermal stability of lithium hexafluorophosphate (LiPF6)-based electrolytes . This leads to improved cycling performance, especially at high temperatures .
Action Environment
The action of this compound is influenced by environmental factors such as temperature. The compound shows improved performance at high temperatures (60 °C), indicating its stability and efficacy under these conditions . It also contributes to the non-flammability of the electrolytes, enhancing the safety of the batteries .
Preparation Methods
Synthetic Routes and Reaction Conditions: Tris(2,2,2-trifluoroethyl) phosphate can be synthesized through a selective three-step substitution transesterification process. This method involves the reaction of this compound with different alcohols in the presence of bases such as DBU (1,8-Diazabicyclo[5.4.0]undec-7-ene) or lithium alkoxides .
Industrial Production Methods: The industrial production of this compound typically involves the reaction of phosphorus oxychloride with 2,2,2-trifluoroethanol under controlled conditions. The reaction is carried out in the presence of a base to neutralize the hydrochloric acid formed during the process .
Chemical Reactions Analysis
Types of Reactions:
Substitution Reactions: Tris(2,2,2-trifluoroethyl) phosphate undergoes substitution reactions where the trifluoroethoxy groups can be replaced by other alkoxy groups.
Common Reagents and Conditions:
Bases: DBU, lithium alkoxides
Solvents: Organic solvents like dichloromethane or tetrahydrofuran
Temperature: Reactions are typically carried out at room temperature or slightly elevated temperatures.
Major Products Formed:
Substitution Reactions: Mixed unsymmetric phosphate triesters
Hydrolysis: Phosphoric acid and 2,2,2-trifluoroethanol
Scientific Research Applications
Chemistry: Tris(2,2,2-trifluoroethyl) phosphate is used as a ligand in organometallic chemistry to enhance labialization in metal clusters and as a substitutional equivalent for carbon monoxide .
Biology and Medicine: The compound is being explored for its potential use in biological systems due to its stability and unique properties. specific applications in biology and medicine are still under research .
Industry:
Electrolyte Additive: It is used as an electrolyte additive to improve the electrochemical performance and as a co-solvent for nonflammable electrolytes in lithium-ion batteries.
Flame Retardant: Due to its non-flammable nature, this compound is used as a flame retardant in various industrial applications.
Comparison with Similar Compounds
- Tris(trimethylsilyl) phosphate
- Tris(pentafluorophenyl)phosphine
- Bis(2,2,2-trifluoroethyl) methylphosphonate
- Tris(1,1,1,3,3,3-hexafluoro-2-propyl) phosphite
Uniqueness: Tris(2,2,2-trifluoroethyl) phosphate stands out due to its high thermal stability and non-flammability, making it particularly valuable in applications requiring these properties. Its ability to act as a ligand in organometallic chemistry and as an electrolyte additive in lithium-ion batteries further distinguishes it from similar compounds .
Properties
IUPAC Name |
tris(2,2,2-trifluoroethyl) phosphate | |
---|---|---|
Source | PubChem | |
URL | https://pubchem.ncbi.nlm.nih.gov | |
Description | Data deposited in or computed by PubChem | |
InChI |
InChI=1S/C6H6F9O4P/c7-4(8,9)1-17-20(16,18-2-5(10,11)12)19-3-6(13,14)15/h1-3H2 | |
Source | PubChem | |
URL | https://pubchem.ncbi.nlm.nih.gov | |
Description | Data deposited in or computed by PubChem | |
InChI Key |
ZMQDTYVODWKHNT-UHFFFAOYSA-N | |
Source | PubChem | |
URL | https://pubchem.ncbi.nlm.nih.gov | |
Description | Data deposited in or computed by PubChem | |
Canonical SMILES |
C(C(F)(F)F)OP(=O)(OCC(F)(F)F)OCC(F)(F)F | |
Source | PubChem | |
URL | https://pubchem.ncbi.nlm.nih.gov | |
Description | Data deposited in or computed by PubChem | |
Molecular Formula |
C6H6F9O4P | |
Source | PubChem | |
URL | https://pubchem.ncbi.nlm.nih.gov | |
Description | Data deposited in or computed by PubChem | |
DSSTOX Substance ID |
DTXSID90307499 | |
Record name | tris(2,2,2-trifluoroethyl) phosphate | |
Source | EPA DSSTox | |
URL | https://comptox.epa.gov/dashboard/DTXSID90307499 | |
Description | DSSTox provides a high quality public chemistry resource for supporting improved predictive toxicology. | |
Molecular Weight |
344.07 g/mol | |
Source | PubChem | |
URL | https://pubchem.ncbi.nlm.nih.gov | |
Description | Data deposited in or computed by PubChem | |
CAS No. |
358-63-4 | |
Record name | 358-63-4 | |
Source | DTP/NCI | |
URL | https://dtp.cancer.gov/dtpstandard/servlet/dwindex?searchtype=NSC&outputformat=html&searchlist=191836 | |
Description | The NCI Development Therapeutics Program (DTP) provides services and resources to the academic and private-sector research communities worldwide to facilitate the discovery and development of new cancer therapeutic agents. | |
Explanation | Unless otherwise indicated, all text within NCI products is free of copyright and may be reused without our permission. Credit the National Cancer Institute as the source. | |
Record name | tris(2,2,2-trifluoroethyl) phosphate | |
Source | EPA DSSTox | |
URL | https://comptox.epa.gov/dashboard/DTXSID90307499 | |
Description | DSSTox provides a high quality public chemistry resource for supporting improved predictive toxicology. | |
Record name | Tris(2,2,2-Trifluoroethyl) phosphate | |
Source | European Chemicals Agency (ECHA) | |
URL | https://echa.europa.eu/information-on-chemicals | |
Description | The European Chemicals Agency (ECHA) is an agency of the European Union which is the driving force among regulatory authorities in implementing the EU's groundbreaking chemicals legislation for the benefit of human health and the environment as well as for innovation and competitiveness. | |
Explanation | Use of the information, documents and data from the ECHA website is subject to the terms and conditions of this Legal Notice, and subject to other binding limitations provided for under applicable law, the information, documents and data made available on the ECHA website may be reproduced, distributed and/or used, totally or in part, for non-commercial purposes provided that ECHA is acknowledged as the source: "Source: European Chemicals Agency, http://echa.europa.eu/". Such acknowledgement must be included in each copy of the material. ECHA permits and encourages organisations and individuals to create links to the ECHA website under the following cumulative conditions: Links can only be made to webpages that provide a link to the Legal Notice page. | |
Retrosynthesis Analysis
AI-Powered Synthesis Planning: Our tool employs the Template_relevance Pistachio, Template_relevance Bkms_metabolic, Template_relevance Pistachio_ringbreaker, Template_relevance Reaxys, Template_relevance Reaxys_biocatalysis model, leveraging a vast database of chemical reactions to predict feasible synthetic routes.
One-Step Synthesis Focus: Specifically designed for one-step synthesis, it provides concise and direct routes for your target compounds, streamlining the synthesis process.
Accurate Predictions: Utilizing the extensive PISTACHIO, BKMS_METABOLIC, PISTACHIO_RINGBREAKER, REAXYS, REAXYS_BIOCATALYSIS database, our tool offers high-accuracy predictions, reflecting the latest in chemical research and data.
Strategy Settings
Precursor scoring | Relevance Heuristic |
---|---|
Min. plausibility | 0.01 |
Model | Template_relevance |
Template Set | Pistachio/Bkms_metabolic/Pistachio_ringbreaker/Reaxys/Reaxys_biocatalysis |
Top-N result to add to graph | 6 |
Feasible Synthetic Routes
Q1: What is Tris(2,2,2-trifluoroethyl) phosphate (TFEP) and what is its primary application in energy storage?
A1: this compound (TFEP) is a fluorinated organophosphate compound being investigated as a safer alternative to conventional flammable electrolytes in lithium-ion batteries [, , ]. Its flame-retardant properties make it a promising candidate for enhancing battery safety.
Q2: How does the addition of TFEP affect the flammability of conventional lithium-ion battery electrolytes?
A2: TFEP acts as a flame retardant by suppressing combustion reactions within the electrolyte [, , ]. Studies have shown that electrolytes containing a TFEP concentration above 20% exhibit non-flammable characteristics [].
Q3: Does the incorporation of TFEP impact the electrochemical performance of lithium-ion batteries?
A3: While TFEP significantly improves safety, it can negatively impact performance. It tends to lower ionic conductivity and discharge capacity compared to conventional electrolytes []. This trade-off necessitates optimization of TFEP concentration and electrolyte formulation.
Q4: What is the impact of TFEP on the thermal stability of charged electrodes like graphite and LixCoO2?
A4: Research suggests that TFEP, while an excellent flame retardant, doesn't necessarily enhance the inherent thermal stability of electrodes like charged graphite and LixCoO2 []. In fact, it may even lead to violent exothermic reactions at the anode side [].
Q5: How does TFEP compare to other flame-retardant additives like trimethyl phosphate in terms of its impact on electrode stability?
A5: Trimethyl phosphate has been observed to improve the thermal stability of cathodes, while TFEP's impact is less straightforward []. This highlights the importance of careful selection and investigation of flame-retardant additives for specific battery chemistries.
Q6: What is the molecular formula and weight of TFEP?
A6: The molecular formula of TFEP is C6H6F9O4P and its molecular weight is 338.06 g/mol.
Q7: How does the structure of TFEP contribute to its flame-retardant properties?
A7: The presence of fluorine atoms in the alkyl substituents of the phosphate group contributes to the flame-retardant nature of TFEP. Fluorine substitution generally increases the oxidative stability and reduces the flammability of organic molecules [].
Q8: Does TFEP interact directly with the electrodes in a lithium-ion battery?
A8: Yes, TFEP participates in the formation of the solid electrolyte interphase (SEI) on electrode surfaces, particularly on the graphite anode [, ]. This SEI layer plays a crucial role in the performance and stability of the battery.
Q9: How does the solvation structure of lithium ions in TFEP-based electrolytes differ from that in conventional electrolytes?
A9: TFEP exhibits weak electron pair-donating ability, leading to the formation of contact ion pairs (Li+···TFSA-) in TFEP-based electrolytes, unlike the solvent-separated ion pairs typically observed in conventional carbonate-based electrolytes [, , ].
Q10: Can the performance of TFEP-based electrolytes be improved by modifying their composition?
A10: Yes, studies have shown that adding co-solvents like ethylene carbonate (EC) or acetonitrile (AN) to TFEP-based electrolytes can improve ionic conductivity and facilitate lithium-ion insertion/deinsertion at the electrodes [, ].
Q11: What is the role of additives like fluoroethylene carbonate in TFEP-containing electrolytes?
A11: Additives like fluoroethylene carbonate (FEC) are used to enhance the compatibility of TFEP-based electrolytes with the graphite anode by promoting the formation of a stable SEI layer [].
Q12: What are the potential advantages of using TFEP as a co-solvent in electrolytes for high-voltage cathodes like LiNi0.5Mn1.5O4?
A12: TFEP's high oxidation stability makes it suitable for high-voltage cathodes []. Additionally, its flame-retardant properties can enhance the safety of batteries operating at higher voltages.
Q13: Does TFEP decompose at high voltages, and if so, what are the implications for battery performance?
A13: TFEP can decompose at high voltages, leading to the formation of byproducts that may negatively impact battery performance. Studies have identified the decomposition products and explored strategies to mitigate this issue [].
Q14: How do the properties of TFEP-based electrolytes affect the performance of lithium-ion batteries at different temperatures?
A14: TFEP's relatively high viscosity can lead to lower ionic conductivity, particularly at low temperatures. This can limit the performance of batteries operating in cold conditions [, ].
Q15: Have TFEP-based electrolytes been tested in practical lithium-ion battery cells?
A15: Yes, researchers have demonstrated the feasibility of using TFEP-based electrolytes in full lithium-ion battery cells, achieving promising cycling performance and safety characteristics [, , ].
Q16: Are there any computational studies investigating the behavior of TFEP in lithium-ion battery electrolytes?
A16: Yes, computational methods like Density Functional Theory (DFT) calculations and Molecular Dynamics (MD) simulations have been employed to study the interactions of TFEP with lithium ions and other electrolyte components [, , ]. These simulations help understand the solvation structure, transport properties, and interfacial behavior of TFEP-based electrolytes at the molecular level.
Q17: What is the impact of the molecular bulkiness of TFEP on its performance in lithium-ion battery electrolytes?
A17: The relatively large size of the TFEP molecule can hinder ionic conductivity by increasing the viscosity of the electrolyte [, ]. This highlights the importance of balancing flame-retardant properties with transport properties when designing electrolytes.
Q18: Can the solvation structure of lithium ions in TFEP-based electrolytes be tuned to improve battery performance?
A18: Yes, research suggests that the solvation structure of lithium ions in TFEP-based electrolytes can be modified by adding co-solvents or additives with different dielectric constants and donating abilities [, , ]. This tuning can impact the ionic conductivity, SEI formation, and overall cell performance.
Q19: What are the key considerations for the practical application of TFEP-based electrolytes in lithium-ion batteries?
A19: Key considerations include balancing the trade-off between safety and electrochemical performance, optimizing electrolyte composition for specific battery chemistries and operating conditions, and understanding the long-term stability and aging characteristics of TFEP-based electrolytes.
Q20: Has the use of TFEP in lithium-ion battery electrolytes been patented?
A20: Yes, there are patents related to the use of TFEP in lithium-ion battery electrolytes, covering specific formulations and applications []. These patents highlight the commercial interest and potential of this technology.
Q21: What are the environmental implications of using TFEP in lithium-ion batteries?
A22: While TFEP offers safety benefits, its environmental impact requires careful assessment. Research into its biodegradability, potential toxicity, and safe disposal methods is crucial to ensure environmentally responsible application [, ].
Q22: Are there any alternative non-flammable electrolytes being explored for lithium-ion batteries?
A23: Yes, research on non-flammable electrolytes extends beyond TFEP. Ionic liquids, solid-state electrolytes, and other flame-retardant additives are being actively investigated as potential alternatives [].
Q23: What is the future outlook for the use of TFEP in lithium-ion batteries?
A23: Continued research into TFEP-based electrolytes, focusing on optimizing composition, enhancing performance, and understanding long-term behavior, is crucial for its successful implementation. If the challenges associated with cost and performance trade-offs can be overcome, TFEP holds significant promise for enabling safer and more energy-dense lithium-ion batteries for a wide range of applications.
Q24: What are the challenges associated with synthesizing TFEP on a large scale?
A24: Scaling up the synthesis of TFEP while maintaining its purity and controlling its cost can be challenging. Optimizing reaction conditions, finding readily available starting materials, and developing efficient purification methods are crucial aspects of large-scale production.
Q25: How can the research community contribute to the development and deployment of safer lithium-ion batteries using TFEP-based electrolytes?
A25: Collaborative efforts involving academia, industry, and government agencies are essential to advance TFEP-based electrolyte research. This includes sharing knowledge, developing standardized testing protocols, and establishing clear safety regulations to accelerate the adoption of this promising technology.
Q26: What are some of the key milestones in the research and development of TFEP for lithium-ion batteries?
A27: Early research focused on understanding the fundamental properties of TFEP and its behavior in electrolyte solutions [, ]. Subsequent studies explored its compatibility with different electrode materials [, , ] and investigated strategies for performance enhancement [, , ]. More recently, research has shifted towards practical demonstrations in full cells and the development of commercially viable formulations [, , ].
Q27: What are some examples of cross-disciplinary collaborations that have contributed to the advancement of TFEP-based electrolytes?
A28: The development of TFEP-based electrolytes has benefited from collaborations between chemists, material scientists, and engineers. For instance, synthetic chemists have developed new routes for TFEP synthesis [, ], while electrochemists have investigated its performance in batteries [, , ]. Material scientists have contributed by analyzing the SEI formation and interfacial phenomena [, , ]. These interdisciplinary efforts are crucial for a comprehensive understanding and successful implementation of TFEP-based electrolytes in next-generation lithium-ion batteries.
Disclaimer and Information on In-Vitro Research Products
Please be aware that all articles and product information presented on BenchChem are intended solely for informational purposes. The products available for purchase on BenchChem are specifically designed for in-vitro studies, which are conducted outside of living organisms. In-vitro studies, derived from the Latin term "in glass," involve experiments performed in controlled laboratory settings using cells or tissues. It is important to note that these products are not categorized as medicines or drugs, and they have not received approval from the FDA for the prevention, treatment, or cure of any medical condition, ailment, or disease. We must emphasize that any form of bodily introduction of these products into humans or animals is strictly prohibited by law. It is essential to adhere to these guidelines to ensure compliance with legal and ethical standards in research and experimentation.