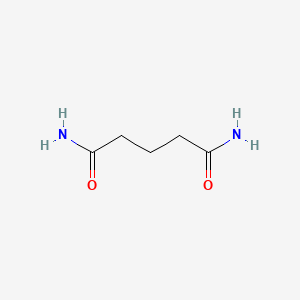
Pentanediamide
Overview
Description
Pentanediamide refers to a class of organic compounds characterized by a five-carbon diamide backbone. A prominent derivative, 2-(4-Ethoxyphenyl sulphonamido) this compound (Fig. 1 in ), has emerged as a novel antitumor and antiangiogenic drug candidate. Developed by S. Sen et al., this compound exhibits selective cytotoxicity against cancer cells while demonstrating negligible toxicity in normal Vero cell lines compared to doxorubicin .
Preparation Methods
Chemical Synthesis from 1,5-Pentanediamine
One of the primary chemical routes to pentanediamide involves the transformation of 1,5-pentanediamine, a diamine precursor, into this compound derivatives. A notable related process is the synthesis of 1,5-pentanediisocyanate from 1,5-pentanediamine, which shares mechanistic insights relevant to this compound preparation.
Process Overview:
- Step 1: Dissolution of 1,5-pentanediamine in an inert solvent under controlled temperature (0–80 °C).
- Step 2: Introduction of phosgene and hydrogen chloride gas under low pressure (0.1–0.2 MPa) for 4–36 hours, facilitating a cold light gasification reaction.
- Step 3: Continued phosgene addition with heating to 150–200 °C under increased pressure (0.2–0.4 MPa) for 8–20 hours to drive the reaction towards the desired product.
- Step 4: Cooling to room temperature, removal of residual gases, and solvent evaporation under vacuum.
- Step 5: Purification by rectification to obtain high-purity this compound or related derivatives.
Key Advantages:
- Controlled molar ratios of phosgene, HCl, and 1,5-pentanediamine reduce undesired side products such as urea and tar resins.
- High yield and improved purification efficiency due to optimized reaction conditions.
Step | Conditions | Purpose |
---|---|---|
S1 | 0–80 °C, inert solvent | Dissolution and uniform mixing |
S2 | 0.1–0.2 MPa, 4–36 h, phosgene + HCl | Cold light gasification reaction stage |
S3 | 150–200 °C, 0.2–0.4 MPa, 8–20 h | Conversion to this compound derivatives |
S4 | Room temperature | Removal of residual gases |
S5 | Vacuum evaporation and rectification | Purification of final product |
This method is detailed in patent CN116239502B and is recognized for its efficiency and scalability in industrial settings.
Fermentation-Based Production of Pentanediamine (Precursor to this compound)
An alternative biotechnological approach involves the fermentation production of pentanediamine, which can subsequently be converted chemically to this compound.
Fermentation Process:
- Culturing genetically engineered cells expressing lysine decarboxylase to convert lysine into pentanediamine.
- The fermentation broth typically contains pentanediamine concentrations exceeding 30 g/L, with optimized strains achieving over 120 g/L.
- Removal of carbon dioxide from the fermentation broth prior to chemical extraction improves yield and reduces alkali consumption during downstream processing.
Extraction and Purification:
- After fermentation, pentanediamine is extracted from the broth.
- Carbon dioxide stripping (via depressurization and heating) is performed to increase pH and facilitate efficient extraction.
- Further chemical conversion steps can then be applied to synthesize this compound.
Parameter | Typical Value |
---|---|
Pentanediamine concentration | >30 g/L to >120 g/L (optimized) |
Carbon dioxide removal | Depressurization and heating |
pH adjustment | After CO2 removal, reduced alkali usage |
This fermentation method is described in patent WO2017198147A1 and represents a sustainable and scalable route for pentanediamine production, enabling subsequent this compound synthesis.
Stability and Pharmaceutical Formulations
Research into this compound derivatives, such as N,N'-bis-[2-(1H-imidazol-4-yl)ethyl]this compound, demonstrates the importance of preparation methods that ensure long-term stability and bioactivity for pharmaceutical applications.
- Stable compositions for oral administration have been developed, showing antiviral activity and long shelf life (up to 10 years).
- These formulations combine this compound derivatives with other active compounds to enhance therapeutic efficacy.
- Preparation methods focus on ensuring chemical stability during storage and ease of industrial scale-up.
This is detailed in patent RU2746692C1, emphasizing preparation methods that maintain compound integrity and efficacy over extended periods.
Summary Table of Preparation Methods for this compound
Chemical Reactions Analysis
Types of Reactions
Pentanediamide undergoes various chemical reactions, including:
Oxidation: this compound can be oxidized to form corresponding carboxylic acids.
Reduction: Reduction of this compound typically yields the corresponding amines.
Substitution: The amide groups in this compound can participate in nucleophilic substitution reactions.
Common Reagents and Conditions
Oxidation: Common oxidizing agents include potassium permanganate (KMnO4) and chromium trioxide (CrO3).
Reduction: Reducing agents such as lithium aluminum hydride (LiAlH4) are often used.
Substitution: Reagents like alkyl halides and conditions involving basic or acidic catalysts are employed.
Major Products
Oxidation: Produces carboxylic acids.
Reduction: Yields primary amines.
Substitution: Results in substituted amides depending on the reagents used.
Scientific Research Applications
Medicinal Applications
1.1 Antimicrobial Properties
Pentanediamide is primarily recognized for its use as an antimicrobial agent . It has been effectively utilized in the treatment of various infections, including:
- Pneumocystis pneumonia (PCP) : Approved by the FDA, pentamidine isethionate is used for treating and preventing PCP in patients with HIV/AIDS. Its mechanism involves inhibition of nucleic acid synthesis in the pathogen Pneumocystis carinii .
- Leishmaniasis : Pentamidine has shown efficacy against Leishmania species, which cause leishmaniasis. Its use here is significant due to the increasing resistance to conventional therapies .
1.2 Mechanism of Action
The pharmacodynamics of this compound involve interference with the synthesis of DNA, RNA, phospholipids, and proteins in target organisms. This broad-spectrum activity makes it a valuable compound in treating protozoal infections .
Environmental Applications
2.1 Metal Extraction
Recent studies have highlighted the use of this compound as an extractant for rare earth elements (REEs) . Its application in solvent extraction processes has shown promising results:
- Selective Recovery : this compound-based extractants have demonstrated high selectivity for extracting Dy(III) and Nd(III) from aqueous solutions, which is crucial for recycling rare metals from electronic waste .
- Efficiency : The efficiency of this compound in extracting these metals can be attributed to its unique molecular structure, which facilitates strong interactions with metal ions .
Case Studies
3.1 Study on Antimicrobial Efficacy
A clinical study assessed the effectiveness of pentamidine in treating PCP among HIV-positive patients. The results indicated a significant reduction in infection rates when administered early in the treatment regimen, showcasing its critical role in managing opportunistic infections .
3.2 Research on Metal Recovery
In a recent research project, this compound was tested for its ability to recover rare earth elements from industrial waste streams. The findings revealed that using this compound as an extractant resulted in recovery rates exceeding 90%, demonstrating its potential for sustainable practices in metal recycling .
Table 1: Comparative Efficacy of Pentamidine Against Various Pathogens
Pathogen | Disease | Efficacy (%) | Dosage (mg) |
---|---|---|---|
Pneumocystis carinii | PCP | 85 | 4-6 |
Leishmania spp. | Leishmaniasis | 75 | 2-4 |
Trypanosoma brucei | Sleeping sickness | 80 | 3-5 |
Table 2: Extraction Efficiency of this compound for Rare Earth Elements
Element | Recovery Rate (%) | Concentration (mg/L) |
---|---|---|
Dy(III) | 92 | 100 |
Nd(III) | 90 | 150 |
Mechanism of Action
The mechanism by which pentanediamide exerts its effects involves its interaction with specific molecular targets. In biological systems, this compound can inhibit certain enzymes by binding to their active sites, thereby interfering with their normal function. This inhibition can lead to a cascade of biochemical events, ultimately affecting cellular processes such as DNA synthesis, protein production, and metabolic pathways .
Comparison with Similar Compounds
Pharmacokinetic Profile :
- Oral Bioavailability : 73.2% in Sprague-Dawley (SD) rats.
- Half-Life : 2.585 ± 0.357 hours post-oral administration.
- Methodology : Validated LC-MS bioanalytical method with protein precipitation (Method 2: serum dilution + acetonitrile) achieving superior recovery (≥85%) and sensitivity (LLOQ: 10 ng/ml) .
Its high oral absorption and bioavailability position it as a viable candidate for oral anticancer therapy .
Cationic Peptidomimetic Lipids (N5 and N6)
Structure :
- N5 : N1,N5-di((Z)-octadec-9-en-1-yl)pentanediamide.
- N6: Extended structure with additional aminoethylaminohexanamide branches .
Applications : siRNA delivery via electrostatic complexation (N/P ratio 1–6).
Performance :
- Particle Size : 135.4–250.7 nm (optimal for cellular uptake).
- Cytotoxicity : >50% cell viability at high concentrations (vs. Lipofectamine 2000).
- Transfection Efficiency : 79–80% GFP expression in BV2/N2a cells (N3 lipid) .
Comparison: Unlike 2-(4-Ethoxyphenyl...) this compound, N5/N6 lack antitumor activity but excel in gene delivery due to their cationic lipid architecture. No pharmacokinetic data are reported.
Alkanediamide-Linked Bisbenzamidines (TH-701)
Structure : Bisbenzamidines connected via this compound .
Activity :
- Antiparasitic: Potent against Trypanosoma brucei and Pneumocystis carinii (IC₅₀ < 1 µM).
- Cytotoxicity: Low toxicity in A549 human lung carcinoma cells .
Comparison: While sharing the this compound linker, TH-701 targets parasitic infections rather than cancer. Structural benzamidine groups enable DNA minor-groove binding, diverging from the sulfonamido moiety in 2-(4-Ethoxyphenyl...) this compound.
Sulfonamido this compound Antitumor Analogs
Structure : Derivatives of 2-(4-acetamidophenylsulfonamido) this compound .
Activity :
- In Vitro : Efficacy against MCF-7 (breast), K-562 (leukemia), and HT-29 (colon) cell lines.
- In Vivo: Inhibition of Ehrlich Ascites Carcinoma (EAC) in mice .
Comparison : These analogs share the sulfonamido-pentanediamide core with 2-(4-Ethoxyphenyl...) this compound but vary in substituents, affecting target selectivity. Pharmacokinetic data remain unreported.
Other this compound Derivatives
- Chemical Intermediates: Examples include N,N′-bis(3-aminopropyl)this compound (CAS: 98502-81-9), used in synthesis but lacking pharmacological data .
Data Tables
Table 1: Pharmacological Comparison of this compound Derivatives
Table 2: Pharmacokinetics of 2-(4-Ethoxyphenyl...) this compound
Biological Activity
Pentanediamide, also known as cadaverine, is a naturally occurring polyamine with significant biological activity across various fields, including medicinal chemistry, biochemistry, and environmental science. This article provides a comprehensive overview of the biological activity of this compound, focusing on its synthesis, mechanisms of action, and potential therapeutic applications.
Overview of this compound
This compound is a straight-chain aliphatic diamine with two amino groups separated by three carbon atoms. It is produced through the decarboxylation of lysine and plays crucial roles in cellular function and metabolism. Its structural formula is represented as follows:
Mechanisms of Biological Activity
This compound exhibits various biological activities, primarily attributed to its role in cell signaling and regulation. The following mechanisms are noteworthy:
- Cell Proliferation : this compound is involved in the regulation of cell growth and differentiation. It acts as a substrate for the synthesis of polyamines, which are essential for DNA stabilization and cellular proliferation.
- Antimicrobial Properties : Studies have shown that this compound derivatives possess antimicrobial activity against a range of pathogens. This property is particularly relevant in the development of new antibiotics.
- Anticancer Activity : Research indicates that this compound derivatives can inhibit specific enzymes involved in cancer cell metabolism, such as S-adenosyl-l-homocysteine hydrolase (SAHase). This inhibition can lead to reduced tumor growth and proliferation.
Synthesis of this compound Derivatives
Recent studies have focused on synthesizing novel this compound derivatives to enhance their biological activities. The following table summarizes some synthesized derivatives along with their biological evaluations:
Compound Name | Synthesis Method | Biological Activity |
---|---|---|
1,5-Pentanediamine Derivative A | Reaction with acyl chlorides | SAHase inhibitor |
1,5-Pentanediamine Derivative B | Alkylation with different alkyl groups | Antimicrobial activity |
1,5-Pentanediamine Derivative C | Condensation reactions | Anticancer properties |
Case Study 1: Anticancer Activity
A study evaluated the anticancer effects of several this compound derivatives against various cancer cell lines, including MCF-7 (breast cancer) and K-562 (leukemia). The results indicated that specific derivatives exhibited significant cytotoxicity compared to control groups. The mechanism was linked to the inhibition of SAHase, leading to altered methylation processes within the cells .
Case Study 2: Antimicrobial Effects
Another investigation focused on the antimicrobial properties of this compound derivatives against Gram-positive and Gram-negative bacteria. The study demonstrated that certain derivatives had a minimum inhibitory concentration (MIC) lower than that of conventional antibiotics, suggesting their potential as novel antimicrobial agents .
Research Findings
Recent research has highlighted several important findings regarding this compound:
- Inhibition of Enzymatic Activity : this compound derivatives have been shown to effectively inhibit SAHase, which is implicated in various diseases including cancer .
- Environmental Applications : this compound's role as a biodegradable compound has made it an attractive candidate for applications in environmental biotechnology, particularly in wastewater treatment processes .
- Potential Drug Development : The diverse biological activities of this compound derivatives position them as promising candidates for drug development targeting various diseases .
Q & A
Basic Research Questions
Q. What are the standard protocols for synthesizing Pentanediamide derivatives, and how can purity be optimized during synthesis?
- Methodological Answer : Solid-phase peptide synthesis (SPPS) is commonly employed, with careful control of reaction conditions (e.g., temperature, solvent polarity, and coupling reagents). To optimize purity, use high-performance liquid chromatography (HPLC) for purification and mass spectrometry (MS) for validation. Ensure batch-to-batch consistency by documenting reaction parameters and employing in-line analytical tools like FTIR for real-time monitoring .
Q. Which spectroscopic techniques are most effective for characterizing this compound’s molecular structure?
- Methodological Answer : Nuclear magnetic resonance (NMR) spectroscopy (¹H/¹³C) resolves functional groups and stereochemistry, while infrared (IR) spectroscopy confirms amide bond formation. Mass spectrometry (MS) validates molecular weight and fragmentation patterns. For reproducibility, adhere to standardized protocols for sample preparation (e.g., deuterated solvents for NMR) and report spectral metrics (e.g., δ values, signal-to-noise ratios) with precision .
Q. How can researchers determine key physicochemical properties of this compound, such as solubility and thermal stability?
- Methodological Answer : Use the shake-flask method to measure solubility in varying pH buffers, coupled with UV-Vis spectrophotometry for quantification. Differential scanning calorimetry (DSC) assesses thermal stability by identifying melting points and decomposition temperatures. Report results with statistical analysis (e.g., mean ± SD) and adhere to metric system conventions (e.g., mL, °C) .
Advanced Research Questions
Q. How should researchers address contradictions in spectral data during structural elucidation of this compound analogs?
- Methodological Answer : Cross-validate results using complementary techniques (e.g., X-ray crystallography for crystalline derivatives or 2D-NMR for complex stereochemistry). Investigate potential impurities via HPLC-MS and replicate experiments under controlled conditions. For unresolved discrepancies, conduct a systematic literature review to identify precedent anomalies or solvent-specific artifacts .
Q. What strategies can optimize reaction yields for novel this compound derivatives with bulky substituents?
- Methodological Answer : Employ design of experiments (DoE) to test variables like catalyst loading, solvent polarity, and reaction time. Use computational tools (e.g., density functional theory, DFT) to predict steric hindrance effects. Monitor intermediates via thin-layer chromatography (TLC) and optimize purification using gradient elution in HPLC .
Q. What methodologies are recommended to investigate this compound’s role in biological systems (e.g., enzyme inhibition)?
- Methodological Answer : Use surface plasmon resonance (SPR) or isothermal titration calorimetry (ITC) to quantify binding affinities. For in vitro assays, validate activity with dose-response curves and include controls for batch variability (e.g., peptide content analysis via amino acid analysis). Ensure statistical rigor by reporting p-values and confidence intervals .
Q. How can computational modeling predict this compound’s interactions with biomolecular targets?
- Methodological Answer : Apply molecular dynamics (MD) simulations to explore conformational flexibility and docking studies (e.g., AutoDock Vina) to identify binding poses. Validate predictions with experimental data (e.g., mutagenesis studies or competitive binding assays). Document force field parameters and solvent models to ensure reproducibility .
Q. What experimental designs are suitable for studying this compound’s stability under varying environmental conditions?
- Methodological Answer : Conduct accelerated stability studies by exposing samples to controlled stressors (e.g., heat, light, humidity). Analyze degradation products via LC-MS and quantify kinetics using Arrhenius plots. For statistical validity, use triplicate samples and report degradation rates with error margins .
Q. Key Considerations for Methodological Rigor
- Data Precision : Report numerical data to reflect instrumental precision (e.g., HPLC purity as 99.2% ± 0.3%) and avoid overreporting insignificant digits .
- Reproducibility : Document equipment specifications (e.g., NMR field strength) and reagent sources (e.g., Sigma-Aldridge catalog numbers) .
- Contradiction Analysis : Use peer-reviewed literature to contextualize anomalous results and refine hypotheses iteratively .
Properties
IUPAC Name |
pentanediamide | |
---|---|---|
Source | PubChem | |
URL | https://pubchem.ncbi.nlm.nih.gov | |
Description | Data deposited in or computed by PubChem | |
InChI |
InChI=1S/C5H10N2O2/c6-4(8)2-1-3-5(7)9/h1-3H2,(H2,6,8)(H2,7,9) | |
Source | PubChem | |
URL | https://pubchem.ncbi.nlm.nih.gov | |
Description | Data deposited in or computed by PubChem | |
InChI Key |
RCCYSVYHULFYHE-UHFFFAOYSA-N | |
Source | PubChem | |
URL | https://pubchem.ncbi.nlm.nih.gov | |
Description | Data deposited in or computed by PubChem | |
Canonical SMILES |
C(CC(=O)N)CC(=O)N | |
Source | PubChem | |
URL | https://pubchem.ncbi.nlm.nih.gov | |
Description | Data deposited in or computed by PubChem | |
Molecular Formula |
C5H10N2O2 | |
Source | PubChem | |
URL | https://pubchem.ncbi.nlm.nih.gov | |
Description | Data deposited in or computed by PubChem | |
DSSTOX Substance ID |
DTXSID20282184 | |
Record name | Pentanediamide | |
Source | EPA DSSTox | |
URL | https://comptox.epa.gov/dashboard/DTXSID20282184 | |
Description | DSSTox provides a high quality public chemistry resource for supporting improved predictive toxicology. | |
Molecular Weight |
130.15 g/mol | |
Source | PubChem | |
URL | https://pubchem.ncbi.nlm.nih.gov | |
Description | Data deposited in or computed by PubChem | |
CAS No. |
3424-60-0 | |
Record name | Pentanediamide | |
Source | DTP/NCI | |
URL | https://dtp.cancer.gov/dtpstandard/servlet/dwindex?searchtype=NSC&outputformat=html&searchlist=24865 | |
Description | The NCI Development Therapeutics Program (DTP) provides services and resources to the academic and private-sector research communities worldwide to facilitate the discovery and development of new cancer therapeutic agents. | |
Explanation | Unless otherwise indicated, all text within NCI products is free of copyright and may be reused without our permission. Credit the National Cancer Institute as the source. | |
Record name | Pentanediamide | |
Source | EPA DSSTox | |
URL | https://comptox.epa.gov/dashboard/DTXSID20282184 | |
Description | DSSTox provides a high quality public chemistry resource for supporting improved predictive toxicology. | |
Synthesis routes and methods I
Procedure details
Synthesis routes and methods II
Procedure details
Synthesis routes and methods III
Procedure details
Retrosynthesis Analysis
AI-Powered Synthesis Planning: Our tool employs the Template_relevance Pistachio, Template_relevance Bkms_metabolic, Template_relevance Pistachio_ringbreaker, Template_relevance Reaxys, Template_relevance Reaxys_biocatalysis model, leveraging a vast database of chemical reactions to predict feasible synthetic routes.
One-Step Synthesis Focus: Specifically designed for one-step synthesis, it provides concise and direct routes for your target compounds, streamlining the synthesis process.
Accurate Predictions: Utilizing the extensive PISTACHIO, BKMS_METABOLIC, PISTACHIO_RINGBREAKER, REAXYS, REAXYS_BIOCATALYSIS database, our tool offers high-accuracy predictions, reflecting the latest in chemical research and data.
Strategy Settings
Precursor scoring | Relevance Heuristic |
---|---|
Min. plausibility | 0.01 |
Model | Template_relevance |
Template Set | Pistachio/Bkms_metabolic/Pistachio_ringbreaker/Reaxys/Reaxys_biocatalysis |
Top-N result to add to graph | 6 |
Feasible Synthetic Routes
Disclaimer and Information on In-Vitro Research Products
Please be aware that all articles and product information presented on BenchChem are intended solely for informational purposes. The products available for purchase on BenchChem are specifically designed for in-vitro studies, which are conducted outside of living organisms. In-vitro studies, derived from the Latin term "in glass," involve experiments performed in controlled laboratory settings using cells or tissues. It is important to note that these products are not categorized as medicines or drugs, and they have not received approval from the FDA for the prevention, treatment, or cure of any medical condition, ailment, or disease. We must emphasize that any form of bodily introduction of these products into humans or animals is strictly prohibited by law. It is essential to adhere to these guidelines to ensure compliance with legal and ethical standards in research and experimentation.