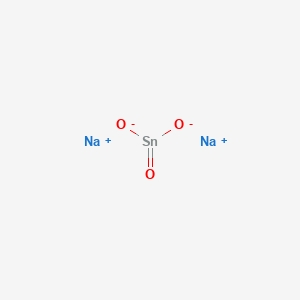
Sodium stannate
Overview
Description
Sodium stannate, formally sodium hexahydroxostannate (IV), is an inorganic compound with the formula Na2 [Sn (OH)6]. This colorless salt forms upon dissolving metallic tin or tin (IV) oxide in sodium hydroxide, and is used as a stabilizer for hydrogen peroxide .
Synthesis Analysis
Sodium stannate can be produced by neutralizing stannic chloride by alkali, obtaining precipitate and washing by water, solid-liquid separation, and obtaining stannic acid. Then, the sodium stannate is synthesized by using the stannic acid and sodium hydroxide, concentrating, crystallizing, filtering, drying and crushing the sodium stannate, and obtaining the product of sodium stannate .Molecular Structure Analysis
Sodium stannate has a molecular formula of Na2SnO3·3H2O . In older literature, stannates are sometimes represented as having the simple oxyanion SnO 32−, in which case this compound is sometimes named as sodium stannate–3–water and represented as Na2SnO3·3H2O .Chemical Reactions Analysis
Sodium stannate is a colorless hexagonal plate-like crystal or white powder. It is soluble in water, the aqueous solution is alkaline, and the relative density of the aqueous solution is 1.438. It is insoluble in alcohol and acetone. The water of crystallization was lost when heated to 140 °C to an anhydrate. In case of acid, it is decomposed into sodium salt and tin dioxide .Physical And Chemical Properties Analysis
Sodium stannate is a colorless hexagonal plate-like crystal or white powder. It is soluble in water, the aqueous solution is alkaline, and the relative density of the aqueous solution is 1.438. It is insoluble in alcohol and acetone. The water of crystallization was lost when heated to 140 °C to an anhydrate .Scientific Research Applications
Electroplating
Sodium stannate is highly desirable in the field of electroplating . It is used as a tin-plating chemical for electroplating processes, which provides a smooth and uniform coating of tin on the substrate.
Tin Alloy Production
Sodium stannate plays a crucial role in the production of tin alloys . These alloys are used in a variety of applications, including soldering and the production of corrosion-resistant equipment.
Solid Superbase Catalysts
Sodium stannate is used in the creation of solid superbase catalysts for dehydrogenation . These catalysts are used in chemical reactions that involve the removal of hydrogen atoms from a molecule.
Flame Retardants
Sodium stannate is used in the production of flame retardants . These substances inhibit or resist the spread of fire in materials.
Solid Electrolytes and Electrode Materials
Sodium stannate is used as solid electrolytes and electrode materials in chemical sources of electrical energy . This application is particularly relevant in the development of advanced battery technologies.
Anodes in Lithium-Ion and Sodium-Ion Batteries
Binary metal oxide stannate (M2SnO4; M = Zn, Mn, Co, etc.) structures, with their high theoretical capacity, superior lithium storage mechanism and suitable operating voltage, as well as their dual suitability for lithium-ion batteries (LIBs) and sodium-ion batteries (SIBs), are strong candidates for next-generation anode materials .
Synthesis of Lanthanum Stannate and Neodymium Stannate Nanoparticles Sodium stannate trihydrate can be used as a precursor to synthesize Lanthanum stannate nanoparticles that are used for the modification of glassy carbon electrodes. It can also be used to synthesize Neodymium stannate nanoparticles for the selective detection of dimetridazole in biological samples .
Mechanism of Action
The most likely mechanism for the promoting effect of Na2SnO3 is the in situ deposition of metallic Sn particles on the Al surface, which act as local inhibitors of the repassivation of Al . The storage of lithium ions in M2SnO4 materials (M2SnO4; M = Zn, Mn, Co, etc.) is carried out by both a conversion reaction mechanism and an alloying–dealloying mechanism .
Safety and Hazards
Future Directions
Binary metal oxide stannate (M2SnO4; M = Zn, Mn, Co, etc.) structures, with their high theoretical capacity, superior lithium storage mechanism and suitable operating voltage, as well as their dual suitability for lithium-ion batteries (LIBs) and sodium-ion batteries (SIBs), are strong candidates for next-generation anode materials .
properties
IUPAC Name |
disodium;dioxido(oxo)tin | |
---|---|---|
Source | PubChem | |
URL | https://pubchem.ncbi.nlm.nih.gov | |
Description | Data deposited in or computed by PubChem | |
InChI |
InChI=1S/2Na.3O.Sn/q2*+1;;2*-1; | |
Source | PubChem | |
URL | https://pubchem.ncbi.nlm.nih.gov | |
Description | Data deposited in or computed by PubChem | |
InChI Key |
TVQLLNFANZSCGY-UHFFFAOYSA-N | |
Source | PubChem | |
URL | https://pubchem.ncbi.nlm.nih.gov | |
Description | Data deposited in or computed by PubChem | |
Canonical SMILES |
[O-][Sn](=O)[O-].[Na+].[Na+] | |
Source | PubChem | |
URL | https://pubchem.ncbi.nlm.nih.gov | |
Description | Data deposited in or computed by PubChem | |
Molecular Formula |
Na2O3Sn | |
Source | PubChem | |
URL | https://pubchem.ncbi.nlm.nih.gov | |
Description | Data deposited in or computed by PubChem | |
Molecular Weight |
212.69 g/mol | |
Source | PubChem | |
URL | https://pubchem.ncbi.nlm.nih.gov | |
Description | Data deposited in or computed by PubChem | |
Physical Description |
Trihydrate: Colorless or white solid; Gradually decomposed by air; [Merck Index] White to off-white crystals and powder; Odorless; Slowly decomposed by air to the oxide; [GFS Chemicals MSDS] | |
Record name | Sodium stannate | |
Source | Haz-Map, Information on Hazardous Chemicals and Occupational Diseases | |
URL | https://haz-map.com/Agents/17364 | |
Description | Haz-Map® is an occupational health database designed for health and safety professionals and for consumers seeking information about the adverse effects of workplace exposures to chemical and biological agents. | |
Explanation | Copyright (c) 2022 Haz-Map(R). All rights reserved. Unless otherwise indicated, all materials from Haz-Map are copyrighted by Haz-Map(R). No part of these materials, either text or image may be used for any purpose other than for personal use. Therefore, reproduction, modification, storage in a retrieval system or retransmission, in any form or by any means, electronic, mechanical or otherwise, for reasons other than personal use, is strictly prohibited without prior written permission. | |
Product Name |
Sodium stannate | |
CAS RN |
12058-66-1 | |
Record name | Sodium stannate | |
Source | ChemIDplus | |
URL | https://pubchem.ncbi.nlm.nih.gov/substance/?source=chemidplus&sourceid=0012058661 | |
Description | ChemIDplus is a free, web search system that provides access to the structure and nomenclature authority files used for the identification of chemical substances cited in National Library of Medicine (NLM) databases, including the TOXNET system. | |
Record name | Stannate (SnO32-), sodium (1:2) | |
Source | EPA Chemicals under the TSCA | |
URL | https://www.epa.gov/chemicals-under-tsca | |
Description | EPA Chemicals under the Toxic Substances Control Act (TSCA) collection contains information on chemicals and their regulations under TSCA, including non-confidential content from the TSCA Chemical Substance Inventory and Chemical Data Reporting. | |
Record name | Disodium tin trioxide | |
Source | European Chemicals Agency (ECHA) | |
URL | https://echa.europa.eu/substance-information/-/substanceinfo/100.031.833 | |
Description | The European Chemicals Agency (ECHA) is an agency of the European Union which is the driving force among regulatory authorities in implementing the EU's groundbreaking chemicals legislation for the benefit of human health and the environment as well as for innovation and competitiveness. | |
Explanation | Use of the information, documents and data from the ECHA website is subject to the terms and conditions of this Legal Notice, and subject to other binding limitations provided for under applicable law, the information, documents and data made available on the ECHA website may be reproduced, distributed and/or used, totally or in part, for non-commercial purposes provided that ECHA is acknowledged as the source: "Source: European Chemicals Agency, http://echa.europa.eu/". Such acknowledgement must be included in each copy of the material. ECHA permits and encourages organisations and individuals to create links to the ECHA website under the following cumulative conditions: Links can only be made to webpages that provide a link to the Legal Notice page. | |
Record name | SODIUM STANNATE ANHYDROUS | |
Source | FDA Global Substance Registration System (GSRS) | |
URL | https://gsrs.ncats.nih.gov/ginas/app/beta/substances/JC8111ZN4W | |
Description | The FDA Global Substance Registration System (GSRS) enables the efficient and accurate exchange of information on what substances are in regulated products. Instead of relying on names, which vary across regulatory domains, countries, and regions, the GSRS knowledge base makes it possible for substances to be defined by standardized, scientific descriptions. | |
Explanation | Unless otherwise noted, the contents of the FDA website (www.fda.gov), both text and graphics, are not copyrighted. They are in the public domain and may be republished, reprinted and otherwise used freely by anyone without the need to obtain permission from FDA. Credit to the U.S. Food and Drug Administration as the source is appreciated but not required. | |
Synthesis routes and methods
Procedure details
Disclaimer and Information on In-Vitro Research Products
Please be aware that all articles and product information presented on BenchChem are intended solely for informational purposes. The products available for purchase on BenchChem are specifically designed for in-vitro studies, which are conducted outside of living organisms. In-vitro studies, derived from the Latin term "in glass," involve experiments performed in controlled laboratory settings using cells or tissues. It is important to note that these products are not categorized as medicines or drugs, and they have not received approval from the FDA for the prevention, treatment, or cure of any medical condition, ailment, or disease. We must emphasize that any form of bodily introduction of these products into humans or animals is strictly prohibited by law. It is essential to adhere to these guidelines to ensure compliance with legal and ethical standards in research and experimentation.