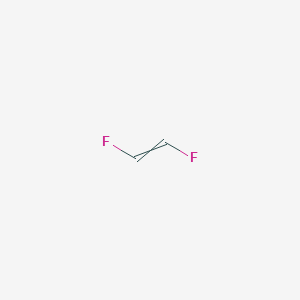
1,2-Difluoroethene
Overview
Description
1,2-Difluoroethene (C₂H₂F₂) is a fluorinated alkene with two fluorine atoms on adjacent carbons. It exists as two stereoisomers: cis-1,2-difluoroethene and trans-1,2-difluoroethene. This compound is synthesized via dehydrochlorination of precursors like 2-chloro-1,2-difluoroethane (HCFC-142) or 1-chloro-1,2-difluoroethane (HCFC-142a) using metal catalysts (Fe, Mg, Pd, etc.) . Its applications span industrial chemistry, including use as a precursor for fluorinated polymers and in liquid crystal displays (LCDs) due to its dielectric properties .
Preparation Methods
Early Synthetic Routes (1950s–1970s)
The first documented synthesis of 1,2-difluoroethene dates to 1955, where it was isolated as a byproduct during the reaction of diborane (B₂H₆) with tetrafluoroethylene (C₂F₄) . While this serendipitous discovery provided initial access to the compound, the yield was insufficient for practical applications.
Trifluoroethylene Dehydrofluorination
In 1957, Haszeldine and Steele developed the first deliberate preparative route using trifluoroethylene (CF₂=CHF) as the starting material . The reaction involved dehydrofluorination under basic conditions, producing this compound alongside hydrogen fluoride (HF). This method achieved moderate yields but required careful control of reaction stoichiometry to minimize polymerization side reactions.
HCFC-132 Dehalogenation
An alternative approach utilized 1,2-dichloro-1,2-difluoroethane (HCFC-132, ClF₂C-CF₂Cl) as a precursor . HCFC-132 was synthesized via the reduction of 1,1,2,2-tetrachloro-1,2-difluoroethane (CFC-112) using lithium aluminum hydride (LiAlH₄) or photoreduction techniques. Subsequent treatment with zinc (Zn) or magnesium (Mg) in ether solvents facilitated the elimination of chlorine atoms, yielding a mixture of E- and Z-1,2-difluoroethene isomers. Fractional distillation at −53.1°C (for E-isomer) and −26.0°C (for Z-isomer) enabled separation .
Industrial and Patent-Based Methods
Catalytic Chlorination-Fluorination
A 2019 patent (CN111116304B) disclosed a two-step gas-phase process for large-scale synthesis :
-
Chlorination : Ethylene (C₂H₄) reacts with chlorine (Cl₂) over a nickel-aluminum (Ni-Al) catalyst (Ni:Al = 45:55 molar ratio) at 250–600°C, producing 1,2-dichloroethane (ClCH₂-CH₂Cl) and 1,1,2-trichloroethane (Cl₂CH-CH₂Cl).
-
Fluorination : The chlorinated intermediates are treated with hydrogen fluoride (HF) using a chromium-copper-zinc (Cr-Cu-Zn) catalyst (Cr:Cu:Zn = 85:5:10) at 200–550°C, yielding this compound and 1,1,2-trifluoroethane.
This method boasts advantages in raw material accessibility (ethylene, chlorine, HF) and scalability, though isomer control remains challenging .
Alternative Patent Approaches
While five additional synthetic routes are referenced in patent literature , details remain proprietary. Indirect evidence suggests explorations into:
-
Electrochemical fluorination of chloroethylenes.
-
Metathesis reactions involving fluorinated alkenes and transition metal catalysts.
Deuteration and Isomer-Specific Synthesis
Stereospecific Deuteration
Treatment of E- or Z-1,2-difluoroethene with sodium deuteroxide (NaOD) in deuterated water (D₂O) at 90–120°C for 48 hours produces deuterated analogues (CDF=CDF) with >95% isotopic purity . This method preserves the stereochemistry of the starting material, enabling applications in kinetic isotope effect studies.
Isomer Separation and Characterization
The E- and Z-isomers exhibit distinct physical and chemical properties:
Property | E-1,2-Difluoroethene | Z-1,2-Difluoroethene |
---|---|---|
Boiling Point (°C) | −53.1 | −26.0 |
Dipole Moment (D) | 0.0 | 2.42 |
(kJ/mol) | −238.3 ± 1.2 | −235.9 ± 1.2 |
Separation is achieved via low-temperature fractional distillation, with purity confirmed by NMR spectroscopy .
Reaction Mechanisms and Byproduct Analysis
Radical Pathways
The Zn/Mg-mediated dehalogenation of HCFC-132 proceeds through a radical chain mechanism :
-
Initiation : Metal reduction generates chloride radicals ().
-
Propagation : abstracts hydrogen from HCFC-132, forming .
-
Termination : Radical recombination releases and .
Side products include trace amounts of 1,1-difluoroethene () and oligomeric fluorocarbons.
Acid-Catalyzed Isomerization
Under acidic conditions (e.g., HF or ), E- and Z-isomers interconvert via a planar carbocation intermediate . This complicates isomer-specific synthesis unless stringent pH control is maintained.
Chemical Reactions Analysis
1,2-Difluoroethene undergoes various chemical reactions, including:
Addition Reactions: It can participate in addition reactions with halogens, hydrogen halides, and other electrophiles.
Polymerization: This compound can be polymerized to form poly(1,2-difluoroethylene), which has applications in the production of fluoropolymers.
Oxidation and Reduction: While specific oxidation and reduction reactions are less common, this compound can be involved in radical reactions under appropriate conditions.
Scientific Research Applications
Applications in Refrigeration
1,2-Difluoroethene has gained attention as a refrigerant due to its favorable thermodynamic properties:
- Low Global Warming Potential (GWP) : As an alternative to hydrofluorocarbons (HFCs), HFO-1132 has a significantly lower GWP, making it an environmentally friendly option for refrigeration systems .
- Refrigerant Properties : Its low boiling point allows for efficient heat absorption during refrigeration cycles, enhancing cooling performance in various applications .
Chemical Synthesis and Polymerization
The compound serves as a crucial building block in the synthesis of various fluorinated polymers:
- Monomer for Fluoropolymers : this compound can be polymerized to create fluorinated polymers that exhibit high thermal stability and chemical resistance. These materials are used in applications ranging from coatings to advanced materials .
- Intermediate in Chemical Reactions : It acts as an intermediate in the synthesis of other fluorinated compounds, expanding its utility in organic chemistry .
Case Studies and Research Findings
Several studies have highlighted the versatility of this compound:
- Refrigerant Performance Evaluation : Research demonstrated that HFO-1132 exhibits comparable performance to traditional refrigerants while reducing environmental impact. A study showed that it performs efficiently in both vapor-compression and absorption refrigeration systems .
- Polymerization Studies : Investigations into the polymerization of this compound revealed that it can produce polymers with unique properties suitable for high-performance applications. For instance, polymers derived from this compound exhibited enhanced resistance to solvents and thermal degradation compared to non-fluorinated counterparts .
Mechanism of Action
The mechanism of action of 1,2-difluoroethene in chemical reactions involves its ability to act as an electrophile or nucleophile, depending on the reaction conditions. The presence of fluorine atoms increases the compound’s reactivity by influencing the electron density around the carbon-carbon double bond . This makes it a versatile reagent in various chemical transformations.
Comparison with Similar Compounds
Comparison with Structurally Similar Compounds
Isomeric Comparisons: cis- vs. trans-1,2-Difluoroethene
- Stability : The cis isomer is thermodynamically more stable than the trans isomer. This "cis effect" arises from conjugative destabilization in the trans configuration, where fluorine atoms oppose each other, disrupting π-bond conjugation. Theoretical calculations using improved virtual orbital (IVO) methods confirm this trend, aligning with principles of maximum hardness and minimum electrophilicity .
- Geometric Clustering : Hierarchical clustering based on cosine distances (0.042 between cis and trans isomers vs. 0.09858 between cis/trans and 1,1-difluoroethene) shows closer geometric similarity between the 1,2-isomers .
- Synthesis : Both isomers are derived from HCFC-142/142a precursors, but reaction conditions (e.g., catalyst choice) influence stereoselectivity .
Comparison with 1,1-Difluoroethene
Comparison with Dichloro-Difluoroethenes
- 1,2-Dichloro-1,2-difluoroethene (C₂Cl₂F₂): Exhibits similar geometric isomerism but lower polarity due to chlorine’s lower electronegativity. Used in specialty polymers but poses higher environmental persistence .
- Reactivity : Hydrolyzes to difluoroacetic acid (DFA) in environmental conditions, unlike this compound, which resists hydrolysis .
Comparison with Trifluoroethene (C₂HF₃)
- Dissociation Pathways : Trifluoroethene undergoes CF fragment loss (C₂F₂⁺ formation) rather than HF elimination, unlike 1,1-difluoroethene, which favors HF elimination .
- Synthetic Utility : Trifluoroethene is a precursor for high-performance polymers, while this compound is niche in LCD applications .
Key Research Findings
Electronic Effects : Fluorine substitution alters π-bond conjugation. In this compound, cis isomer stability is driven by orbital interactions (IVO calculations show lower electrophilicity for cis) .
Environmental Impact : 1,1-Difluoroethene derivatives (e.g., polyfluorinated carboxylic acids) are detected in water systems at higher concentrations than legacy PFASs, raising concerns about bioaccumulation .
Thermophysical Properties :
- 1,1-Difluoroethene (R1132a) has a speed of sound between 193–383 K (up to 3 MPa) with ±0.3% deviation from Helmholtz-energy models .
- Liquid-phase viscosity correlates linearly with temperature (223–421 K) for R1132a .
Data Tables
Table 1: Thermophysical Properties of 1,1-Difluoroethene (R1132a)
Temperature (K) | Speed of Sound (m/s) | Viscosity (μPa·s) |
---|---|---|
193 | 220 ± 0.5 | – |
298 | 315 ± 0.7 | 12.3 |
383 | 410 ± 1.2 | 18.9 |
Table 2: Stability and Reactivity Trends
Biological Activity
1,2-Difluoroethene (HFO-1132) is a fluorinated organic compound with significant applications in various fields, including refrigeration and as an intermediate in chemical synthesis. Understanding its biological activity is crucial for assessing its environmental impact and potential health effects. This article reviews the biological activity of this compound, highlighting its toxicological profile, metabolic pathways, and relevant case studies.
This compound is characterized by its two fluorine substituents on a two-carbon alkene backbone. Its structure can be represented as:
This compound exists in two geometric isomers: cis and trans , which exhibit different physical and chemical properties.
Acute Toxicity
This compound has low acute toxicity based on inhalation studies conducted in laboratory animals. The reported lethal concentration (LC50) values indicate that it poses minimal risk at typical exposure levels. In studies involving rats and dogs, the compound demonstrated a relatively high safety threshold before adverse effects were observed .
Chronic Exposure and Metabolism
Research indicates that chronic exposure to this compound may lead to metabolic alterations. The primary metabolic pathway involves cytochrome P450 enzymes that facilitate the conversion of the compound into various metabolites. Studies have shown that both isomers can induce different CYP isoforms, which may lead to sex-dependent differences in metabolism and toxicity .
Metabolic Pathways
The metabolism of this compound occurs primarily through oxidation processes mediated by cytochrome P450 enzymes. Key findings include:
- CYP Isoform Induction : Both cis- and trans-1,2-difluoroethene can induce CYP enzymes, although the extent of induction varies between sexes in animal models.
- Metabolite Formation : The major metabolites formed during the metabolism of this compound include difluoroacetic acid and other fluorinated compounds .
Environmental Impact Studies
Several case studies have investigated the environmental persistence and bioaccumulation potential of this compound. For instance:
- Groundwater Contamination : In regions where this compound was used as a refrigerant or solvent, groundwater studies revealed detectable levels of the compound. Remediation efforts often involve advanced oxidation processes to degrade the compound effectively .
- Aquatic Toxicity : Research has shown that this compound exhibits varying degrees of toxicity to aquatic organisms, with LC50 values indicating potential risks to fish populations at elevated concentrations .
Summary of Key Research Data
A comprehensive overview of research findings related to the biological activity of this compound is presented in the table below:
Q & A
Q. Basic: How can geometric isomers (cis/trans) of 1,2-difluoroethene be experimentally distinguished, and what synthesis routes yield specific isomers?
Methodological Answer:
- Synthesis : The cis and trans isomers are synthesized via dehydrohalogenation of 1-chloro-1,2-difluoroethane or thermal cracking of dichlorofluoromethane with hydrogenation catalysts .
- Characterization : Use vibrational spectroscopy (IR/Raman) to identify differences in dipole moments and symmetry. For example, the trans isomer (C2h symmetry) shows distinct stretching modes compared to the cis isomer (Cs symmetry) . Nuclear Overhauser Effect (NOE) in NMR can also differentiate spatial proximity of fluorine atoms in the cis isomer .
Q. Basic: What experimental techniques are used to assess the thermal stability and decomposition pathways of this compound?
Methodological Answer:
- Thermogravimetric Analysis (TGA) : Measure mass loss under controlled heating to identify decomposition thresholds.
- Mass Spectrometry (MS) : Fragment ions (e.g., C2F2<sup>+</sup> or CHF2<sup>+</sup>) reveal dominant pathways like HF elimination or fluorine migration .
- Threshold Photoelectron Spectroscopy : Determines ionization energies and metastable dissociation channels (e.g., adiabatic ionization energy ≈10.14 eV) .
Q. Basic: How is this compound evaluated for applications in lithium-ion battery electrolytes?
Methodological Answer:
- Electrochemical Testing : Compare discharge capacity and cycle life in coin cells using this compound-based electrolytes vs. commercial alternatives.
- Safety Metrics : Assess flammability resistance via accelerated rate calorimetry (ARC) and evaluate compatibility with anode/cathode materials (e.g., LiCoO2) using XPS to detect passivation layers .
Q. Advanced: How do electron correlation effects and localized molecular orbitals influence NMR J-couplings in this compound?
Methodological Answer:
- Localized Molecular Orbital (LMO) Analysis : Partition Fermi Contact (FC), Spin-Dipole (SD), and Paramagnetic Spin-Orbit (PSO) contributions. For example, σ(C–C) bonding orbitals contribute 133 Hz to <sup>1</sup>J(C–C) in trans-1,2-difluoroethene .
- Density Functional Theory (DFT) : Use hybrid functionals (e.g., B3LYP) with exact-exchange corrections to model J-coupling trends. The FC mechanism dominates, but SD/PSO terms are significant for vicinal <sup>3</sup>J(F–F) due to fluorine lone pairs .
Q. Advanced: What mechanistic insights into dissociation dynamics can be gained from studying this compound cations?
Methodological Answer:
- Coincidence Techniques : Measure breakdown diagrams and time-of-flight (TOF) peaks to identify fast vs. metastable dissociation (e.g., loss of CF fragments vs. HF elimination).
- Quantum-Chemical Calculations : Compare experimental appearance energies (e.g., 13.86 eV for C2F2<sup>+</sup>) with G3B3-calculated thresholds to validate reverse barriers in exit channels .
Q. Advanced: How can DFT methods reconcile discrepancies in thermochemical data for this compound reactions?
Methodological Answer:
- Functional Selection : Use hybrid functionals (e.g., B3LYP) with gradient corrections and exact-exchange terms to reduce errors in atomization energies (average deviation <2.4 kcal/mol) .
- Basis Set Benchmarking : Compare CCSD(T)/CBS benchmarks with DFT results for bond dissociation energies (e.g., C–F vs. C–H) to identify systematic biases .
Q. Advanced: What experimental and computational approaches are used to study cis-trans isomerization kinetics in this compound?
Methodological Answer:
- Laser-Induced Temperature-Jump (T-Jump) : Monitor isomerization rates via time-resolved FTIR under non-equilibrium conditions.
- Transition State Theory (TST) : Calculate activation energies (Δ<sup>‡</sup>G) using M06-2X/cc-pVTZ to model π-bond rotation barriers .
Q. Advanced: How are systematic reviews designed to assess potential toxicity of this compound derivatives?
Methodological Answer:
- Inclusion Criteria : Prioritize inhalation/oral exposure studies in mammals (e.g., rodent models) with endpoints like respiratory effects or systemic toxicity .
- Confidence Grading : Upgrade evidence if consistent across species (e.g., mutagenicity in bacterial assays vs. in vivo models) .
Q. Advanced: What strategies optimize copolymer synthesis using this compound for functional materials?
Methodological Answer:
Properties
IUPAC Name |
1,2-difluoroethene | |
---|---|---|
Source | PubChem | |
URL | https://pubchem.ncbi.nlm.nih.gov | |
Description | Data deposited in or computed by PubChem | |
InChI |
InChI=1S/C2H2F2/c3-1-2-4/h1-2H | |
Source | PubChem | |
URL | https://pubchem.ncbi.nlm.nih.gov | |
Description | Data deposited in or computed by PubChem | |
InChI Key |
WFLOTYSKFUPZQB-UHFFFAOYSA-N | |
Source | PubChem | |
URL | https://pubchem.ncbi.nlm.nih.gov | |
Description | Data deposited in or computed by PubChem | |
Canonical SMILES |
C(=CF)F | |
Source | PubChem | |
URL | https://pubchem.ncbi.nlm.nih.gov | |
Description | Data deposited in or computed by PubChem | |
Molecular Formula |
C2H2F2 | |
Source | PubChem | |
URL | https://pubchem.ncbi.nlm.nih.gov | |
Description | Data deposited in or computed by PubChem | |
Related CAS |
38193-40-7 | |
Record name | Ethene, 1,2-difluoro-, homopolymer | |
Source | CAS Common Chemistry | |
URL | https://commonchemistry.cas.org/detail?cas_rn=38193-40-7 | |
Description | CAS Common Chemistry is an open community resource for accessing chemical information. Nearly 500,000 chemical substances from CAS REGISTRY cover areas of community interest, including common and frequently regulated chemicals, and those relevant to high school and undergraduate chemistry classes. This chemical information, curated by our expert scientists, is provided in alignment with our mission as a division of the American Chemical Society. | |
Explanation | The data from CAS Common Chemistry is provided under a CC-BY-NC 4.0 license, unless otherwise stated. | |
DSSTOX Substance ID |
DTXSID80937587 | |
Record name | 1,2-Difluoroethene | |
Source | EPA DSSTox | |
URL | https://comptox.epa.gov/dashboard/DTXSID80937587 | |
Description | DSSTox provides a high quality public chemistry resource for supporting improved predictive toxicology. | |
Molecular Weight |
64.03 g/mol | |
Source | PubChem | |
URL | https://pubchem.ncbi.nlm.nih.gov | |
Description | Data deposited in or computed by PubChem | |
CAS No. |
1691-13-0 | |
Record name | Ethene, 1,2-difluoro- | |
Source | CAS Common Chemistry | |
URL | https://commonchemistry.cas.org/detail?cas_rn=1691-13-0 | |
Description | CAS Common Chemistry is an open community resource for accessing chemical information. Nearly 500,000 chemical substances from CAS REGISTRY cover areas of community interest, including common and frequently regulated chemicals, and those relevant to high school and undergraduate chemistry classes. This chemical information, curated by our expert scientists, is provided in alignment with our mission as a division of the American Chemical Society. | |
Explanation | The data from CAS Common Chemistry is provided under a CC-BY-NC 4.0 license, unless otherwise stated. | |
Record name | 1,2-Fluoroethene | |
Source | ChemIDplus | |
URL | https://pubchem.ncbi.nlm.nih.gov/substance/?source=chemidplus&sourceid=0001691130 | |
Description | ChemIDplus is a free, web search system that provides access to the structure and nomenclature authority files used for the identification of chemical substances cited in National Library of Medicine (NLM) databases, including the TOXNET system. | |
Record name | 1,2-Difluoroethene | |
Source | EPA DSSTox | |
URL | https://comptox.epa.gov/dashboard/DTXSID80937587 | |
Description | DSSTox provides a high quality public chemistry resource for supporting improved predictive toxicology. | |
Record name | 1,2-difluoroethylene | |
Source | European Chemicals Agency (ECHA) | |
URL | https://echa.europa.eu/substance-information/-/substanceinfo/100.015.352 | |
Description | The European Chemicals Agency (ECHA) is an agency of the European Union which is the driving force among regulatory authorities in implementing the EU's groundbreaking chemicals legislation for the benefit of human health and the environment as well as for innovation and competitiveness. | |
Explanation | Use of the information, documents and data from the ECHA website is subject to the terms and conditions of this Legal Notice, and subject to other binding limitations provided for under applicable law, the information, documents and data made available on the ECHA website may be reproduced, distributed and/or used, totally or in part, for non-commercial purposes provided that ECHA is acknowledged as the source: "Source: European Chemicals Agency, http://echa.europa.eu/". Such acknowledgement must be included in each copy of the material. ECHA permits and encourages organisations and individuals to create links to the ECHA website under the following cumulative conditions: Links can only be made to webpages that provide a link to the Legal Notice page. | |
Retrosynthesis Analysis
AI-Powered Synthesis Planning: Our tool employs the Template_relevance Pistachio, Template_relevance Bkms_metabolic, Template_relevance Pistachio_ringbreaker, Template_relevance Reaxys, Template_relevance Reaxys_biocatalysis model, leveraging a vast database of chemical reactions to predict feasible synthetic routes.
One-Step Synthesis Focus: Specifically designed for one-step synthesis, it provides concise and direct routes for your target compounds, streamlining the synthesis process.
Accurate Predictions: Utilizing the extensive PISTACHIO, BKMS_METABOLIC, PISTACHIO_RINGBREAKER, REAXYS, REAXYS_BIOCATALYSIS database, our tool offers high-accuracy predictions, reflecting the latest in chemical research and data.
Strategy Settings
Precursor scoring | Relevance Heuristic |
---|---|
Min. plausibility | 0.01 |
Model | Template_relevance |
Template Set | Pistachio/Bkms_metabolic/Pistachio_ringbreaker/Reaxys/Reaxys_biocatalysis |
Top-N result to add to graph | 6 |
Feasible Synthetic Routes
Disclaimer and Information on In-Vitro Research Products
Please be aware that all articles and product information presented on BenchChem are intended solely for informational purposes. The products available for purchase on BenchChem are specifically designed for in-vitro studies, which are conducted outside of living organisms. In-vitro studies, derived from the Latin term "in glass," involve experiments performed in controlled laboratory settings using cells or tissues. It is important to note that these products are not categorized as medicines or drugs, and they have not received approval from the FDA for the prevention, treatment, or cure of any medical condition, ailment, or disease. We must emphasize that any form of bodily introduction of these products into humans or animals is strictly prohibited by law. It is essential to adhere to these guidelines to ensure compliance with legal and ethical standards in research and experimentation.