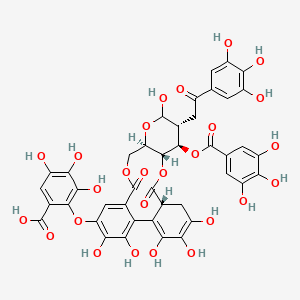
Rugosin B
- Click on QUICK INQUIRY to receive a quote from our team of experts.
- With the quality product at a COMPETITIVE price, you can focus more on your research.
Overview
Description
Rugosin B is a naturally occurring compound with diverse biological activities reported across multiple studies. It is classified as:
- An antimicrobial peptide: Isolated from the skin of the frog Rana rugosa, this compound consists of 33 amino acid residues and exhibits broad-spectrum activity against Gram-positive and Gram-negative bacteria (e.g., E. coli NIHJ and S. aureus 209P) .
Preparation Methods
Overview of Rugosin B Synthesis Approaches
Two primary approaches dominate the preparation of this compound:
- Chemoenzymatic, biomimetic total synthesis : This approach mimics natural biosynthetic pathways using enzymatic catalysis combined with chemical synthesis steps.
- Chemical total synthesis via organic transformations : This involves stepwise organic reactions including protection/deprotection, coupling, oxidation, and cyclization to build the complex ellagitannin structure.
Chemoenzymatic, Biomimetic Synthesis of this compound
A significant advancement in the synthesis of this compound was reported using a chemoenzymatic approach. This method involves:
- Starting from anthraquinone derivatives as monomeric units.
- Enzymatic dimerization to form heterodimeric this compound.
- Few-step synthesis (3-4 steps) enabling efficient assembly of the complex molecule.
This biomimetic strategy supports the hypothesis of dimerization of substituted monomeric intermediates in natural biosynthesis of (+)-rugulosin B and C, providing a concise and selective route to this compound and related analogues.
Organic Synthesis Strategies for this compound
Building Block Preparation and Key Reactions
The chemical synthesis of this compound involves the preparation of key building blocks such as galloylated glucose derivatives and their subsequent functionalization:
- Galloylation and protection : Starting from glucose derivatives, selective galloylation is performed using 3,4,5-tri-O-benzylgallic acid chloride, prepared via oxalyl chloride activation.
- Phenolic protection : Benzyl ethers are used to protect phenolic hydroxyl groups, facilitating selective reactions.
- Formation of orthoquinone intermediates : These intermediates are crucial for subsequent coupling reactions.
Example synthesis steps include:
Step | Reactants & Conditions | Outcome | Yield (%) |
---|---|---|---|
Galloylation of glucose derivative with benzyl-protected gallic acid chloride | Use of oxalyl chloride, DMF catalyst, toluene solvent, 70 °C, 45 min | Formation of tri-galloylated glucose intermediate | High, ~92% |
Benzylation of phenols | NaH, BnBr in DMF/toluene, RT, 2 h | Benzyl ether protected intermediates | ~92% |
Reduction | Diisobutylaluminium hydride in CH2Cl2, 0 °C, 1 h | Conversion to desired alcohol intermediates | ~100% |
These steps set the stage for the critical coupling and cyclization reactions.
Coupling and Cyclization
- Oxa-Michael addition : Phenolic nucleophiles add to orthoquinone intermediates, followed by elimination and reductive aromatization to form diaryl ether linkages.
- Ullmann coupling : Used for C–O digallate formation, although efficiency can be limited depending on protection groups.
- Intramolecular aryl–aryl coupling : Enables formation of hexahydroxydiphenoyl (HHDP) groups, crucial for ellagitannin structure.
These steps are essential for constructing the complex polyphenolic framework of this compound.
Detailed Preparation Method from Patent Literature
A patented method provides a multi-step synthetic route involving:
- Hydrolysis of precursor compounds in mixed organic solvent and water under alkaline conditions to yield intermediate compounds.
- Synthesis of key intermediates (e.g., compound G) via hydrogenation using Pd/C catalyst under mild pressure and temperature.
- Final coupling with methoxycarbamates (phenyl or nitrophenyl methoxycarbamate) in organic solvents such as toluene or acetonitrile, with bases like triethylamine, at 55-75 °C for 20 hours.
- Post-reaction workup includes cooling, filtration, washing, drying, and recrystallization to obtain this compound with high purity (>99%) and yield (~92%).
Step | Reagents & Conditions | Notes | Yield (%) | Purity (%) |
---|---|---|---|---|
Hydrolysis of compound A to B | Alkali, mixed organic solvent/water | Controlled pH and temperature | Not specified | - |
Hydrogenation of compound F to G | 5% Pd/C, H2, 30 °C, 0.3 MPa, 2 h | Filtration and pH adjustment | 92.32 | 98.54 |
Coupling to form this compound | Phenyl methoxycarbamate, TEA, toluene, 75 °C, 20 h | Cooling and recrystallization | 92.36 | 99.57 |
This method emphasizes careful control of reaction parameters and purification steps to achieve high-quality this compound.
Summary Table of this compound Preparation Methods
Methodology | Key Features | Advantages | Limitations |
---|---|---|---|
Chemoenzymatic biomimetic synthesis | Enzymatic dimerization, anthraquinone precursors, 3-4 steps | Biomimetic, efficient, selective | Requires enzyme availability and optimization |
Chemical total synthesis | Multi-step organic synthesis, protection/deprotection, coupling | Well-established, scalable | Complex, time-consuming, requires multiple purifications |
Patent method | Hydrolysis, hydrogenation, carbamate coupling | High yield and purity, detailed process | Requires specific reagents and conditions |
Research Findings and Implications
- The chemoenzymatic approach offers a promising biomimetic route that may simplify this compound synthesis and provide insights into its biosynthesis.
- Organic synthesis methods provide versatile routes to analogues and derivatives, enabling structure-activity relationship studies.
- The patented synthetic route demonstrates the feasibility of producing this compound at high purity and yield, suitable for pharmaceutical and research applications.
Chemical Reactions Analysis
Types of Reactions
This compound undergoes various types of chemical reactions, including:
Oxidation: The hydroxyl groups can be oxidized to form ketones or carboxylic acids.
Reduction: The carbonyl groups can be reduced to form alcohols.
Substitution: The aromatic rings can undergo electrophilic or nucleophilic substitution reactions.
Common Reagents and Conditions
Common reagents used in these reactions include:
Oxidizing agents: Potassium permanganate (KMnO₄), chromium trioxide (CrO₃).
Reducing agents: Sodium borohydride (NaBH₄), lithium aluminum hydride (LiAlH₄).
Catalysts: Palladium on carbon (Pd/C), platinum oxide (PtO₂).
Major Products
The major products formed from these reactions depend on the specific conditions and reagents used. For example, oxidation of the hydroxyl groups can yield carboxylic acids, while reduction of the carbonyl groups can produce alcohols.
Scientific Research Applications
Antioxidant Activity
Rugosin B exhibits potent antioxidant properties, which are crucial for preventing oxidative stress-related diseases.
- Scavenging Activity : Studies have demonstrated that this compound has a strong scavenging ability against free radicals such as DPPH and superoxide anions. Its IC50 values for these activities are significantly lower than those of common antioxidants like quercetin and ascorbic acid, indicating its superior efficacy .
- Mechanism : The antioxidant activity is attributed to the presence of multiple hydroxyl groups in its structure, which facilitate electron donation to neutralize free radicals .
Inhibition of HMG-CoA Reductase
This compound has been shown to inhibit HMG-CoA reductase (HMGR), an important enzyme in cholesterol biosynthesis.
- Inhibitory Potency : The IC50 value for this compound's inhibition of HMGR was reported at 1.46 µM, making it one of the more potent inhibitors among tested compounds .
- Clinical Relevance : This property suggests potential applications in managing hyperlipidemia and cardiovascular diseases by lowering cholesterol levels.
Antibacterial Properties
Recent studies have highlighted the antibacterial potential of this compound against drug-resistant bacteria such as Methicillin-resistant Staphylococcus aureus (MRSA).
- Mechanism of Action : this compound acts as an antibacterial sensitizer, enhancing the efficacy of existing antibiotics against resistant strains without exhibiting direct antibacterial activity itself .
- Synergistic Effects : When combined with antibiotics, this compound has been shown to restore sensitivity in MRSA strains, offering a promising strategy for overcoming antibiotic resistance .
Anti-Diabetic Potential
Research indicates that this compound may have applications in diabetes management due to its ability to inhibit digestive enzymes.
- Inhibition of α-Amylase : Studies have demonstrated that extracts containing this compound significantly inhibit α-amylase activity, which is crucial for carbohydrate digestion. This inhibition can help regulate blood glucose levels post-meal .
- Potential Therapeutic Use : These findings suggest that this compound could be developed into a functional food or supplement for diabetes management.
Summary Table of Biological Activities
Mechanism of Action
The mechanism of action of this compound involves its interaction with specific molecular targets and pathways. The hydroxyl and carbonyl groups play a crucial role in its reactivity and ability to form hydrogen bonds with other molecules. These interactions can influence various biological processes and pathways, leading to its observed effects.
Comparison with Similar Compounds
Structural Properties :
- Molecular formula: C₃₅H₅₂O₁₄ (plant-derived) or 33-amino-acid peptide (frog-derived) .
- Key features: Intramolecular disulfide bonds (in the peptide form) or galloyl ester groups (in the tannin form) .
Comparison with Structurally Similar Compounds
Rugosin A
Property | Rugosin B (Plant) | Rugosin A (Plant) |
---|---|---|
Source | Rosa gallica, Stachyurus praecox | Same as this compound |
Molecular Formula | C₃₅H₅₂O₁₄ | C₂₁H₂₈O₁₀ |
Molecular Weight | 1104.762 g/mol | 954.672 g/mol |
Key Activity | DPP-IV inhibition (IC₅₀: ~12 μM) | Moderate DPP-IV inhibition |
Structural Difference | Additional galactose moiety | Lacks galactose substitution |
Functional Insights :
- This compound shows stronger DPP-IV inhibition than Rugosin A, attributed to its galactose group enhancing binding affinity .
Rugosin C
Property | This compound (Frog) | Rugosin C (Frog) |
---|---|---|
Amino Acid Residues | 33 | 37 |
Disulfide Bonds | 1 intramolecular bond | 1 intramolecular bond |
Antimicrobial Spectrum | Broad (Gram+/−) | Narrow (Gram+) |
Homology to Brevinin-2 | 33% | 45% (Rugosin A) / Lower (C) |
Mechanistic Contrast :
- This compound’s lower homology to brevinin-2 correlates with its broader antimicrobial range compared to Rugosin C .
- Both peptides disrupt bacterial membranes, but this compound’s charge distribution enhances efficacy against E. coli .
Comparison with Functionally Similar Compounds
Tellimagrandin II (Tannin)
Property | This compound (Plant) | Tellimagrandin II |
---|---|---|
Source | Rosa gallica | Camellia sinensis |
Activity | DPP-IV inhibition, Anticancer | Antiviral, Antioxidant |
DPP-IV IC₅₀ | ~12 μM | >50 μM |
Structural Feature | Galloyl esters | Hexahydroxydiphenoyl esters |
Key Finding: this compound’s galloyl groups confer superior enzyme inhibition compared to Tellimagrandin II’s diphenoyl structure .
Brevinin-2 (Antimicrobial Peptide)
Property | This compound (Frog) | Brevinin-2 |
---|---|---|
Source | Rana rugosa | Rana porosa brevipoda |
Amino Acid Residues | 33 | 33 |
Homology | 33% | 45% (to Rugosin A) |
Antimicrobial Potency | MIC: 2–8 μg/mL (Gram−) | MIC: 4–16 μg/mL (Gram+) |
Mechanistic Divergence :
- This compound’s cationic residues enhance membrane disruption in Gram-negative bacteria, unlike Brevinin-2’s Gram+ specificity .
Critical Analysis of Divergent Reports
- Nomenclature Conflict: The term "this compound" refers to both a plant-derived tannin and a frog-derived peptide. This duality underscores the need for source-specific characterization in studies .
- Activity Spectrum : The plant-derived form shows metabolic (DPP-IV) and anticancer activities, while the frog-derived form specializes in antimicrobial action .
Q & A
Basic Research Questions
Q. How is Rugosin B structurally characterized, and what key spectroscopic techniques are essential for validation?
this compound (C₂₁H₂₆O₁₀, MW 954.672) is characterized using nuclear magnetic resonance (NMR) for elucidating carbon-hydrogen frameworks, mass spectrometry (MS) for molecular weight confirmation, and X-ray crystallography for stereochemical resolution. Its optical rotation ([α] +124 in ethanol) and purity are validated via high-performance liquid chromatography (HPLC) and elemental analysis. Researchers must cross-reference spectral data with existing literature to confirm structural integrity .
Q. Which plant sources are commonly utilized for this compound extraction, and how do their yields vary?
this compound is primarily isolated from Stachyurus praecox and Rosa rugosa. Yield variations depend on plant tissue (e.g., bark vs. leaves), seasonal harvesting times, and extraction protocols. For example, Stachyurus praecox bark yields higher concentrations (0.12–0.25% dry weight) compared to Rosa rugosa petals (0.08–0.15%) under optimized ethanol-water extraction .
Q. What are the foundational chemical properties of this compound critical for experimental design?
Key properties include:
- Solubility : Polar solvents (e.g., methanol, ethanol) at pH 5.0–6.0.
- Stability : Degrades above 60°C; requires storage at –20°C under nitrogen.
- Spectroscopic signatures : UV-Vis absorption maxima at 280 nm (phenolic groups) and IR bands at 3400 cm⁻¹ (hydroxyl stretches) .
Advanced Research Questions
Q. How can researchers resolve contradictions in reported bioactivity data for this compound (e.g., antioxidant vs. pro-oxidant effects)?
Contradictions arise from assay variability (e.g., DPPH vs. FRAP for antioxidant capacity) and concentration-dependent effects. To address this:
- Standardize protocols : Use identical cell lines (e.g., HepG2 for cytotoxicity assays) and control ROS levels.
- Validate mechanisms : Pair in vitro assays with gene expression analysis (e.g., Nrf2 pathway) to confirm dose-response relationships .
Q. What experimental strategies optimize this compound isolation from complex plant matrices?
Optimization involves:
- Solvent gradients : Ethanol-water (70:30 v/v) at 40°C for maximum solubility.
- Chromatography : Preparative HPLC with C18 columns (acetonitrile/0.1% formic acid mobile phase).
- Species-specific adjustments : Stachyurus praecox requires longer maceration (48 hrs) than Rosa rugosa (24 hrs) due to lignin interference .
Q. How should researchers design assays to investigate this compound’s interaction with cellular targets (e.g., NF-κB or MAPK pathways)?
- In vitro models : Use siRNA knockdown in THP-1 macrophages to isolate pathway-specific effects.
- Dosage ranges : 10–100 μM to avoid off-target cytotoxicity (preliminary MTT assays required).
- Multi-omics integration : Combine transcriptomics (RNA-seq) and proteomics (LC-MS/MS) to map upstream/downstream regulators .
Q. What methodologies validate this compound’s stability under physiological conditions for pharmacokinetic studies?
- Simulated gastric fluid (SGF) assays : Incubate at 37°C for 2 hrs, monitor degradation via LC-MS.
- Plasma stability tests : Use rat plasma (4°C vs. 25°C) to assess half-life.
- Light exposure trials : UV-Vis spectroscopy to detect photodegradation products .
Q. How can computational modeling enhance the understanding of this compound’s structure-activity relationships?
- Molecular docking : AutoDock Vina to predict binding affinities with COX-2 or PI3K.
- QSAR models : Correlate substituent effects (e.g., galactose moiety) with anti-inflammatory IC₅₀ values.
- MD simulations : GROMACS to assess conformational stability in lipid bilayers .
Q. Methodological Considerations
Q. What criteria ensure rigorous formulation of hypotheses in this compound research?
Apply the FINER framework :
- Feasible : Adequate plant biomass and analytical resources.
- Novel : Address gaps (e.g., unexplored synergistic effects with flavonoids).
- Ethical : Compliance with Nagoya Protocol for plant sourcing .
Q. How should researchers document experimental protocols for reproducibility?
Properties
bioactivity |
Antibacterial |
---|---|
sequence |
SLFSLIKAGAKFLGKNLLKQGAQYAACKVSKEC |
Origin of Product |
United States |
Disclaimer and Information on In-Vitro Research Products
Please be aware that all articles and product information presented on BenchChem are intended solely for informational purposes. The products available for purchase on BenchChem are specifically designed for in-vitro studies, which are conducted outside of living organisms. In-vitro studies, derived from the Latin term "in glass," involve experiments performed in controlled laboratory settings using cells or tissues. It is important to note that these products are not categorized as medicines or drugs, and they have not received approval from the FDA for the prevention, treatment, or cure of any medical condition, ailment, or disease. We must emphasize that any form of bodily introduction of these products into humans or animals is strictly prohibited by law. It is essential to adhere to these guidelines to ensure compliance with legal and ethical standards in research and experimentation.