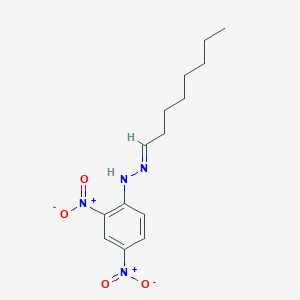
Octanal 2,4-dinitrophenylhydrazone
Overview
Description
Octanal 2,4-dinitrophenylhydrazone: is a derivative of octanal, an aliphatic aldehyde, and 2,4-dinitrophenylhydrazine. This compound is primarily used as an analytical standard in various chemical analyses. It is known for its application in identifying and quantifying carbonyl compounds, particularly aldehydes and ketones, due to its ability to form stable hydrazone derivatives .
Mechanism of Action
Target of Action
Octanal 2,4-dinitrophenylhydrazone, also known as 2,4-dinitro-N-[(E)-octylideneamino]aniline, has been used as an analytical standard to test its efficacy against various bacterial strains . The primary targets of this compound include penicillin-resistant S. pneumoniae (PRSP) , methicillin-resistant Staphylococcus aureus (MRSA) , and other streptococci . These bacteria are significant pathogens in human diseases, and resistance to common antibiotics makes them particularly challenging to treat.
Mode of Action
It’s known that the compound’s efficacy against these bacterial strains has been tested, with its minimum inhibitory concentrations (mic) determined .
Result of Action
This compound has shown efficacy against pneumococcal infections in local infection and mouse sepsis models . This suggests that the compound can lead to the inhibition or death of the targeted bacteria, thereby alleviating the infection.
Preparation Methods
Synthetic Routes and Reaction Conditions: The synthesis of Octanal 2,4-dinitrophenylhydrazone involves the reaction of octanal with 2,4-dinitrophenylhydrazine. The reaction typically occurs in an acidic medium, often using sulfuric acid as a catalyst. The reaction proceeds through a nucleophilic addition-elimination mechanism, where the hydrazine group adds to the carbonyl carbon of octanal, followed by the elimination of water to form the hydrazone derivative .
Industrial Production Methods: Industrial production of 2,4-dinitrophenylhydrazones, including this compound, often involves the use of carbon dioxide pressure to optimize the reaction conditions. This method enhances the yield and purity of the product by controlling the reaction environment .
Chemical Reactions Analysis
Types of Reactions: Octanal 2,4-dinitrophenylhydrazone primarily undergoes condensation reactions. The compound is formed through a condensation reaction between octanal and 2,4-dinitrophenylhydrazine, resulting in the elimination of water .
Common Reagents and Conditions:
Reagents: Octanal, 2,4-dinitrophenylhydrazine, sulfuric acid (catalyst)
Conditions: Acidic medium, typically at room temperature or slightly elevated temperatures
Major Products: The major product of the reaction between octanal and 2,4-dinitrophenylhydrazine is this compound. This compound is characterized by its yellow to orange color and its stability, which makes it suitable for analytical purposes .
Scientific Research Applications
Octanal 2,4-dinitrophenylhydrazone has several applications in scientific research:
Analytical Chemistry: Used as a standard for the detection and quantification of aldehydes and ketones in various samples. .
Environmental Science: Employed in the analysis of environmental samples to detect carbonyl compounds, which are important indicators of air quality and pollution.
Comparison with Similar Compounds
- Nonanal 2,4-dinitrophenylhydrazone
- Hexanal 2,4-dinitrophenylhydrazone
- Butyraldehyde 2,4-dinitrophenylhydrazone
Comparison: Octanal 2,4-dinitrophenylhydrazone is unique due to its specific aliphatic chain length, which influences its physical and chemical properties. Compared to shorter-chain aldehyde derivatives like Hexanal 2,4-dinitrophenylhydrazone, this compound has a higher molecular weight and different solubility characteristics. These differences can affect its behavior in analytical applications and its interaction with various reagents .
Properties
IUPAC Name |
2,4-dinitro-N-[(E)-octylideneamino]aniline | |
---|---|---|
Source | PubChem | |
URL | https://pubchem.ncbi.nlm.nih.gov | |
Description | Data deposited in or computed by PubChem | |
InChI |
InChI=1S/C14H20N4O4/c1-2-3-4-5-6-7-10-15-16-13-9-8-12(17(19)20)11-14(13)18(21)22/h8-11,16H,2-7H2,1H3/b15-10+ | |
Source | PubChem | |
URL | https://pubchem.ncbi.nlm.nih.gov | |
Description | Data deposited in or computed by PubChem | |
InChI Key |
HIPWOUSEWCCFDN-XNTDXEJSSA-N | |
Source | PubChem | |
URL | https://pubchem.ncbi.nlm.nih.gov | |
Description | Data deposited in or computed by PubChem | |
Canonical SMILES |
CCCCCCCC=NNC1=C(C=C(C=C1)[N+](=O)[O-])[N+](=O)[O-] | |
Source | PubChem | |
URL | https://pubchem.ncbi.nlm.nih.gov | |
Description | Data deposited in or computed by PubChem | |
Isomeric SMILES |
CCCCCCC/C=N/NC1=C(C=C(C=C1)[N+](=O)[O-])[N+](=O)[O-] | |
Source | PubChem | |
URL | https://pubchem.ncbi.nlm.nih.gov | |
Description | Data deposited in or computed by PubChem | |
Molecular Formula |
C14H20N4O4 | |
Source | PubChem | |
URL | https://pubchem.ncbi.nlm.nih.gov | |
Description | Data deposited in or computed by PubChem | |
Molecular Weight |
308.33 g/mol | |
Source | PubChem | |
URL | https://pubchem.ncbi.nlm.nih.gov | |
Description | Data deposited in or computed by PubChem | |
CAS No. |
1726-77-8 | |
Record name | NSC402247 | |
Source | DTP/NCI | |
URL | https://dtp.cancer.gov/dtpstandard/servlet/dwindex?searchtype=NSC&outputformat=html&searchlist=402247 | |
Description | The NCI Development Therapeutics Program (DTP) provides services and resources to the academic and private-sector research communities worldwide to facilitate the discovery and development of new cancer therapeutic agents. | |
Explanation | Unless otherwise indicated, all text within NCI products is free of copyright and may be reused without our permission. Credit the National Cancer Institute as the source. | |
Retrosynthesis Analysis
AI-Powered Synthesis Planning: Our tool employs the Template_relevance Pistachio, Template_relevance Bkms_metabolic, Template_relevance Pistachio_ringbreaker, Template_relevance Reaxys, Template_relevance Reaxys_biocatalysis model, leveraging a vast database of chemical reactions to predict feasible synthetic routes.
One-Step Synthesis Focus: Specifically designed for one-step synthesis, it provides concise and direct routes for your target compounds, streamlining the synthesis process.
Accurate Predictions: Utilizing the extensive PISTACHIO, BKMS_METABOLIC, PISTACHIO_RINGBREAKER, REAXYS, REAXYS_BIOCATALYSIS database, our tool offers high-accuracy predictions, reflecting the latest in chemical research and data.
Strategy Settings
Precursor scoring | Relevance Heuristic |
---|---|
Min. plausibility | 0.01 |
Model | Template_relevance |
Template Set | Pistachio/Bkms_metabolic/Pistachio_ringbreaker/Reaxys/Reaxys_biocatalysis |
Top-N result to add to graph | 6 |
Feasible Synthetic Routes
Disclaimer and Information on In-Vitro Research Products
Please be aware that all articles and product information presented on BenchChem are intended solely for informational purposes. The products available for purchase on BenchChem are specifically designed for in-vitro studies, which are conducted outside of living organisms. In-vitro studies, derived from the Latin term "in glass," involve experiments performed in controlled laboratory settings using cells or tissues. It is important to note that these products are not categorized as medicines or drugs, and they have not received approval from the FDA for the prevention, treatment, or cure of any medical condition, ailment, or disease. We must emphasize that any form of bodily introduction of these products into humans or animals is strictly prohibited by law. It is essential to adhere to these guidelines to ensure compliance with legal and ethical standards in research and experimentation.