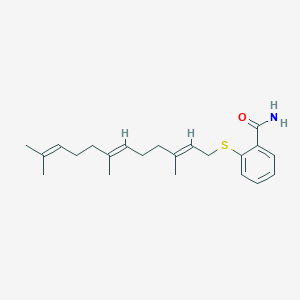
Farnesyl Thiosalicylic Acid Amide
Overview
Description
Farnesyl Thiosalicylic Acid Amide is a derivative of farnesyl thiosalicylic acid. It is known for its ability to inhibit the Ras protein, which is involved in cell growth and differentiation. This compound has shown potential in cancer research due to its ability to reduce Ras-GTP levels and inhibit cell growth .
Mechanism of Action
Target of Action
Farnesyl Thiosalicylic Acid Amide (FTS-A) primarily targets the Ras protein . Ras proteins, including H-RAS, K-RAS, and N-RAS isoforms, are crucial for regulating many malignant cell functions, such as proliferation, differentiation, survival, and induction of angiogenesis . They are activated by mutation in approximately one-third of human cancers .
Mode of Action
FTS-A competitively blocks Ras signaling . It specifically inhibits the activation of Ras by oncogenic stimuli and growth factor receptors, thereby suppressing Ras-dependent tumor growth . FTS-A also interferes with Ras membrane interactions that are crucial for Ras-dependent transformation .
Biochemical Pathways
The primary biochemical pathway affected by FTS-A is the Ras signaling pathway . By inhibiting the activation of Ras, FTS-A disrupts the signaling cascade that leads to the uncontrolled growth and division of cancer cells . The downstream effects of this disruption include the inhibition of cell proliferation and the induction of cell death .
Pharmacokinetics
The pharmacokinetic profile of FTS-A is characterized by slow absorption and a rapid elimination phase following oral administration . The half-life (T1/2) is approximately 3.6 ± 2.2 hours on day 1 . The exposure of FTS-A (Cmax; day 1 AUCinf vs. day 15 AUC0–12h) is similar between days 1 and 15 . These properties impact the bioavailability of FTS-A and determine the dosing regimen for therapeutic applications.
Result of Action
FTS-A reduces Ras-GTP levels and inhibits cell growth . It has been shown to inhibit the growth of PANC-1 and U87 tumor cells with IC50 values of 20 and 10 µM, respectively . In animal models, treatment with FTS-A has been shown to inhibit tumor growth by at least 50% of controls .
Biochemical Analysis
Biochemical Properties
Farnesyl Thiosalicylic Acid Amide acts by reducing the levels of Ras-GTP, thereby inhibiting cell growth . It has been found to be effective against Panc-1 and U87 cells, with IC50 values of 20 and 10 μM respectively . The compound interacts with Ras proteins, dislodging them from the cell membrane and inhibiting downstream signaling .
Cellular Effects
This compound has been shown to have significant effects on various types of cells. In Panc-1 and U87 cells, it reduces the levels of Ras-GTP, leading to inhibition of cell growth . It has also been found to induce apoptosis in HepG2 and Hep3B cells .
Molecular Mechanism
The molecular mechanism of this compound involves its interaction with Ras proteins. It selectively dislodges active Ras proteins from the cell membrane, inhibiting downstream signaling . This results in a reduction of Ras-GTP levels and inhibition of cell growth .
Temporal Effects in Laboratory Settings
While specific studies on the temporal effects of this compound in laboratory settings are limited, it has been observed that the compound induces a dose- and time-dependent decrease of cell growth in HCC cells .
Dosage Effects in Animal Models
In animal models, this compound has been shown to inhibit tumor growth. For example, in nude mice bearing either U87 glioblastoma or PANC-1 tumors, treatment with 100 mg/kg FTS Amide twice daily for four days inhibited tumor growth by at least 50% of controls .
Metabolic Pathways
Given its role as a Ras inhibitor, it is likely to be involved in pathways related to cell growth and proliferation .
Transport and Distribution
Given its mechanism of action, it is likely to interact with the cell membrane where Ras proteins are located .
Subcellular Localization
The subcellular localization of this compound is likely to be at the cell membrane, given its interaction with Ras proteins that are located at the inner surface of the plasma membrane .
Preparation Methods
Synthetic Routes and Reaction Conditions: Farnesyl Thiosalicylic Acid Amide can be synthesized through a multi-step process involving the reaction of farnesyl thiosalicylic acid with an amine. The reaction typically requires a solvent such as dimethyl sulfoxide (DMSO) and may involve heating to facilitate the reaction .
Industrial Production Methods: Industrial production of this compound involves scaling up the laboratory synthesis process. This includes optimizing reaction conditions to maximize yield and purity. The use of continuous flow reactors and advanced purification techniques such as chromatography can enhance the efficiency of production .
Chemical Reactions Analysis
Types of Reactions: Farnesyl Thiosalicylic Acid Amide undergoes various chemical reactions, including:
Oxidation: This reaction can be facilitated by oxidizing agents such as hydrogen peroxide.
Reduction: Reducing agents like sodium borohydride can be used to reduce the compound.
Substitution: Nucleophilic substitution reactions can occur, where nucleophiles replace functional groups in the compound.
Common Reagents and Conditions:
Oxidation: Hydrogen peroxide, under acidic or basic conditions.
Reduction: Sodium borohydride, typically in an alcohol solvent.
Substitution: Nucleophiles such as halides or amines, often in polar aprotic solvents.
Major Products: The major products formed from these reactions depend on the specific reagents and conditions used. For example, oxidation may yield sulfoxides or sulfones, while reduction can produce alcohols or amines .
Scientific Research Applications
Farnesyl Thiosalicylic Acid Amide has a wide range of scientific research applications:
Chemistry: Used as a reagent in organic synthesis and as a model compound for studying reaction mechanisms.
Biology: Investigated for its role in modulating protein interactions and signaling pathways.
Medicine: Explored as a potential therapeutic agent for cancer treatment due to its ability to inhibit Ras-mediated signaling.
Industry: Utilized in the development of new materials and as a component in chemical manufacturing processes
Comparison with Similar Compounds
Farnesyl Thiosalicylic Acid: The parent compound, also known for inhibiting Ras.
Farnesyl Thioacetic Acid: Another derivative with similar inhibitory effects on Ras.
15-deoxy-Δ12,14-prostaglandin J2: A lipid compound that activates TRPA1 channels.
Uniqueness: Farnesyl Thiosalicylic Acid Amide is unique due to its higher potency compared to its parent compound, Farnesyl Thiosalicylic Acid. It has shown greater efficacy in inhibiting tumor cell growth and has a distinct mechanism of action involving the dislodgment of Ras from the cell membrane .
Biological Activity
Farnesyl thiosalicylic acid amide (FTS-A) is a derivative of farnesyl thiosalicylic acid (FTS), which has garnered attention for its potential therapeutic applications, particularly in oncology. This article delves into the biological activity of FTS-A, focusing on its mechanisms of action, efficacy in various cancer models, and relevant research findings.
FTS-A functions primarily as a Ras inhibitor , disrupting the membrane localization of Ras proteins, which are pivotal in cell signaling pathways that promote tumor growth and survival. The compound interferes with the farnesylation process, a post-translational modification essential for Ras function. By inhibiting this process, FTS-A effectively reduces the active forms of Ras proteins (K-Ras and N-Ras) within cancer cells, leading to decreased cell proliferation and increased apoptosis .
In Vitro Studies
In vitro studies have demonstrated that FTS-A exhibits significant cytotoxic effects across various cancer cell lines. Notably, it has been shown to:
- Reduce Cell Viability : In experiments with pancreatic cancer (Panc-1) and glioblastoma (U87) cell lines, FTS-A caused a dose-dependent decrease in cell numbers. The IC50 values ranged from 10 to 20 μM for both cell types .
- Induce Apoptosis : Higher concentrations (above 50 μM) resulted in observable cell death, indicating its potential as an effective chemotherapeutic agent .
In Vivo Studies
FTS-A's efficacy has also been evaluated in vivo using mouse models:
- Tumor Growth Inhibition : In a study involving nude mice implanted with U87 glioblastoma cells, administration of FTS-A (100 mg/kg orally twice daily) significantly inhibited tumor growth compared to control groups. Tumor volume increase was markedly lower in treated mice, suggesting effective therapeutic activity without inducing inflammatory responses .
- Combination Therapy : Research has indicated that combining FTS with other anticancer agents like curcumin enhances its efficacy. This synergistic effect was observed through targeted delivery systems that improved the bioavailability and cytotoxicity of curcumin when used alongside FTS .
Case Studies and Clinical Implications
While no clinical trials specifically targeting FTS-A have been reported, preclinical studies provide a strong foundation for its potential use in cancer therapy. For instance:
- A study on human melanoma xenografts demonstrated that daily doses of FTS led to a concentration-dependent reduction in tumor growth. The combination of FTS with dacarbazine resulted in a significant mean tumor growth reduction of 56% .
- Another investigation highlighted the use of FTS in enhancing the effects of traditional chemotherapy agents, suggesting that it may serve as an adjuvant therapy to improve outcomes in Ras-driven cancers .
Summary of Research Findings
The following table summarizes key findings regarding the biological activity of FTS-A:
Study Type | Cell Line | IC50 (μM) | Effect Observed | Additional Notes |
---|---|---|---|---|
In Vitro | Panc-1 | 10-20 | Dose-dependent decrease in viability | Induces apoptosis at higher concentrations |
In Vitro | U87 | 10-20 | Dose-dependent decrease in viability | Reduces N-Ras-GTP levels |
In Vivo | U87 (mouse model) | N/A | Significant tumor growth inhibition | No inflammatory response noted |
Combination Study | Various | <1.0 | Synergistic effect with curcumin | Enhanced cytotoxicity via PEG micelles |
Properties
IUPAC Name |
2-[(2E,6E)-3,7,11-trimethyldodeca-2,6,10-trienyl]sulfanylbenzamide | |
---|---|---|
Source | PubChem | |
URL | https://pubchem.ncbi.nlm.nih.gov | |
Description | Data deposited in or computed by PubChem | |
InChI |
InChI=1S/C22H31NOS/c1-17(2)9-7-10-18(3)11-8-12-19(4)15-16-25-21-14-6-5-13-20(21)22(23)24/h5-6,9,11,13-15H,7-8,10,12,16H2,1-4H3,(H2,23,24)/b18-11+,19-15+ | |
Source | PubChem | |
URL | https://pubchem.ncbi.nlm.nih.gov | |
Description | Data deposited in or computed by PubChem | |
InChI Key |
GZTMFRUGZMZCRD-CFBAGHHKSA-N | |
Source | PubChem | |
URL | https://pubchem.ncbi.nlm.nih.gov | |
Description | Data deposited in or computed by PubChem | |
Canonical SMILES |
CC(=CCCC(=CCCC(=CCSC1=CC=CC=C1C(=O)N)C)C)C | |
Source | PubChem | |
URL | https://pubchem.ncbi.nlm.nih.gov | |
Description | Data deposited in or computed by PubChem | |
Isomeric SMILES |
CC(=CCC/C(=C/CC/C(=C/CSC1=CC=CC=C1C(=O)N)/C)/C)C | |
Source | PubChem | |
URL | https://pubchem.ncbi.nlm.nih.gov | |
Description | Data deposited in or computed by PubChem | |
Molecular Formula |
C22H31NOS | |
Source | PubChem | |
URL | https://pubchem.ncbi.nlm.nih.gov | |
Description | Data deposited in or computed by PubChem | |
Molecular Weight |
357.6 g/mol | |
Source | PubChem | |
URL | https://pubchem.ncbi.nlm.nih.gov | |
Description | Data deposited in or computed by PubChem | |
Retrosynthesis Analysis
AI-Powered Synthesis Planning: Our tool employs the Template_relevance Pistachio, Template_relevance Bkms_metabolic, Template_relevance Pistachio_ringbreaker, Template_relevance Reaxys, Template_relevance Reaxys_biocatalysis model, leveraging a vast database of chemical reactions to predict feasible synthetic routes.
One-Step Synthesis Focus: Specifically designed for one-step synthesis, it provides concise and direct routes for your target compounds, streamlining the synthesis process.
Accurate Predictions: Utilizing the extensive PISTACHIO, BKMS_METABOLIC, PISTACHIO_RINGBREAKER, REAXYS, REAXYS_BIOCATALYSIS database, our tool offers high-accuracy predictions, reflecting the latest in chemical research and data.
Strategy Settings
Precursor scoring | Relevance Heuristic |
---|---|
Min. plausibility | 0.01 |
Model | Template_relevance |
Template Set | Pistachio/Bkms_metabolic/Pistachio_ringbreaker/Reaxys/Reaxys_biocatalysis |
Top-N result to add to graph | 6 |
Feasible Synthetic Routes
Disclaimer and Information on In-Vitro Research Products
Please be aware that all articles and product information presented on BenchChem are intended solely for informational purposes. The products available for purchase on BenchChem are specifically designed for in-vitro studies, which are conducted outside of living organisms. In-vitro studies, derived from the Latin term "in glass," involve experiments performed in controlled laboratory settings using cells or tissues. It is important to note that these products are not categorized as medicines or drugs, and they have not received approval from the FDA for the prevention, treatment, or cure of any medical condition, ailment, or disease. We must emphasize that any form of bodily introduction of these products into humans or animals is strictly prohibited by law. It is essential to adhere to these guidelines to ensure compliance with legal and ethical standards in research and experimentation.