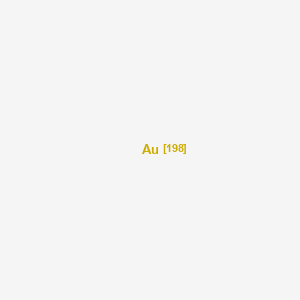
Gold-198
- Click on QUICK INQUIRY to receive a quote from our team of experts.
- With the quality product at a COMPETITIVE price, you can focus more on your research.
Description
Gold-198 is a suspension of sub-micrometre-sized particles of gold in a fluid, typically water or an organic solvent. This compound is notable for its unique optical, electronic, and molecular recognition properties, which have garnered significant interest in various scientific fields. This compound is particularly valued for its applications in medical diagnostics and treatment, especially in the context of cancer therapy due to its radioactive properties .
Preparation Methods
Synthetic Routes and Reaction Conditions: The synthesis of gold (198Au) colloidal typically involves the reduction of gold salts. One common method is the arabinoxylan-mediated synthesis, where an aqueous suspension of arabinoxylan is mixed with a solution of hydrogen tetrachloroaurate (H198AuCl4). This process reduces Au3+ to form gold (198Au) nanoparticles without the need for additional reducing or stabilizing agents .
Industrial Production Methods: Industrial production of gold (198Au) colloidal often involves neutron irradiation of highly purified gold (Au-197) in a nuclear reactor. The gold target is placed in the neutron flux, leading to the formation of gold (198Au) through the reaction:
197Au+n→198Au+γ
Properties
CAS No. |
10043-49-9 |
---|---|
Molecular Formula |
Au |
Molecular Weight |
197.968244 g/mol |
IUPAC Name |
gold-198 |
InChI |
InChI=1S/Au/i1+1 |
InChI Key |
PCHJSUWPFVWCPO-OUBTZVSYSA-N |
SMILES |
[Au] |
Isomeric SMILES |
[198Au] |
Canonical SMILES |
[Au] |
Key on ui other cas no. |
10043-49-9 |
Synonyms |
198Au radioisotope Au-198 radioisotope Gold-198 |
Origin of Product |
United States |
Disclaimer and Information on In-Vitro Research Products
Please be aware that all articles and product information presented on BenchChem are intended solely for informational purposes. The products available for purchase on BenchChem are specifically designed for in-vitro studies, which are conducted outside of living organisms. In-vitro studies, derived from the Latin term "in glass," involve experiments performed in controlled laboratory settings using cells or tissues. It is important to note that these products are not categorized as medicines or drugs, and they have not received approval from the FDA for the prevention, treatment, or cure of any medical condition, ailment, or disease. We must emphasize that any form of bodily introduction of these products into humans or animals is strictly prohibited by law. It is essential to adhere to these guidelines to ensure compliance with legal and ethical standards in research and experimentation.