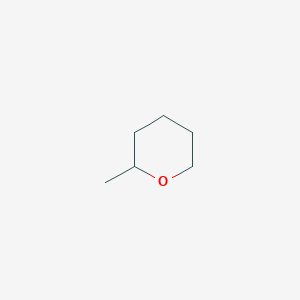
2-Methyltetrahydropyran
Overview
Description
2-Methyltetrahydropyran is an organic compound with the chemical formula C6H12O. It is a colorless liquid with a sweet taste and a weak ether odor . This compound is a derivative of tetrahydropyran, which is a six-membered ring containing five carbon atoms and one oxygen atom.
Preparation Methods
Synthetic Routes and Reaction Conditions: 2-Methyltetrahydropyran can be synthesized through various methods. One common method involves the platinum-catalyzed hydroalkoxylation of γ- and δ-hydroxy olefins . Another method includes the reaction of tertiary 1,4- and 1,5-diols with cerium ammonium nitrate at room temperature, yielding tetrahydropyran derivatives in high yield and stereoselectivity . Additionally, lanthanide triflates can catalyze the intramolecular Markovnikov-type hydroalkoxylation/cyclization of primary/secondary and aliphatic/aromatic hydroxyalkenes in room temperature ionic liquids .
Industrial Production Methods: Industrial production of this compound typically involves the hydrogenation of the 3,4-isomer of dihydropyran using Raney nickel . This method is efficient and yields high purity products suitable for various applications.
Chemical Reactions Analysis
Types of Reactions: 2-Methyltetrahydropyran undergoes several types of chemical reactions, including oxidation, reduction, substitution, and hydroalkoxylation .
Common Reagents and Conditions:
Oxidation: Common oxidizing agents include potassium permanganate and chromium trioxide.
Reduction: Sodium borohydride and lithium aluminum hydride are frequently used reducing agents.
Substitution: Halogenation reactions often use halogenating agents like chlorine or bromine.
Hydroalkoxylation: Catalysts such as platinum, cerium ammonium nitrate, and lanthanide triflates are used.
Major Products: The major products formed from these reactions include various substituted tetrahydropyran derivatives, which can be further utilized in the synthesis of more complex organic molecules .
Scientific Research Applications
2-Methyltetrahydropyran has a wide range of applications in scientific research:
Mechanism of Action
The mechanism of action of 2-Methyltetrahydropyran involves its interaction with various molecular targets and pathways. For example, in reduction reactions, it undergoes stereoselective reduction to yield equatorial alcohols, facilitated by intramolecular assistance from the cyclic ether oxygen . This mechanism highlights the compound’s ability to participate in complex chemical transformations.
Comparison with Similar Compounds
Tetrahydropyran: A six-membered ring with five carbon atoms and one oxygen atom.
2H-Pyran: A structural motif present in many natural products.
2-Methyltetrahydropyran-4-one: A derivative used in various chemical reactions.
Uniqueness: this compound is unique due to its specific structural configuration, which imparts distinct chemical reactivity and stability. Its ability to undergo stereoselective reactions and form stable derivatives makes it valuable in synthetic chemistry and industrial applications .
Properties
IUPAC Name |
2-methyloxane | |
---|---|---|
Source | PubChem | |
URL | https://pubchem.ncbi.nlm.nih.gov | |
Description | Data deposited in or computed by PubChem | |
InChI |
InChI=1S/C6H12O/c1-6-4-2-3-5-7-6/h6H,2-5H2,1H3 | |
Source | PubChem | |
URL | https://pubchem.ncbi.nlm.nih.gov | |
Description | Data deposited in or computed by PubChem | |
InChI Key |
YBDQLHBVNXARAU-UHFFFAOYSA-N | |
Source | PubChem | |
URL | https://pubchem.ncbi.nlm.nih.gov | |
Description | Data deposited in or computed by PubChem | |
Canonical SMILES |
CC1CCCCO1 | |
Source | PubChem | |
URL | https://pubchem.ncbi.nlm.nih.gov | |
Description | Data deposited in or computed by PubChem | |
Molecular Formula |
C6H12O | |
Source | PubChem | |
URL | https://pubchem.ncbi.nlm.nih.gov | |
Description | Data deposited in or computed by PubChem | |
DSSTOX Substance ID |
DTXSID60906150 | |
Record name | 2-Methyloxane | |
Source | EPA DSSTox | |
URL | https://comptox.epa.gov/dashboard/DTXSID60906150 | |
Description | DSSTox provides a high quality public chemistry resource for supporting improved predictive toxicology. | |
Molecular Weight |
100.16 g/mol | |
Source | PubChem | |
URL | https://pubchem.ncbi.nlm.nih.gov | |
Description | Data deposited in or computed by PubChem | |
CAS No. |
10141-72-7 | |
Record name | 2-Methyltetrahydropyran | |
Source | ChemIDplus | |
URL | https://pubchem.ncbi.nlm.nih.gov/substance/?source=chemidplus&sourceid=0010141727 | |
Description | ChemIDplus is a free, web search system that provides access to the structure and nomenclature authority files used for the identification of chemical substances cited in National Library of Medicine (NLM) databases, including the TOXNET system. | |
Record name | 2-Methyloxane | |
Source | EPA DSSTox | |
URL | https://comptox.epa.gov/dashboard/DTXSID60906150 | |
Description | DSSTox provides a high quality public chemistry resource for supporting improved predictive toxicology. | |
Retrosynthesis Analysis
AI-Powered Synthesis Planning: Our tool employs the Template_relevance Pistachio, Template_relevance Bkms_metabolic, Template_relevance Pistachio_ringbreaker, Template_relevance Reaxys, Template_relevance Reaxys_biocatalysis model, leveraging a vast database of chemical reactions to predict feasible synthetic routes.
One-Step Synthesis Focus: Specifically designed for one-step synthesis, it provides concise and direct routes for your target compounds, streamlining the synthesis process.
Accurate Predictions: Utilizing the extensive PISTACHIO, BKMS_METABOLIC, PISTACHIO_RINGBREAKER, REAXYS, REAXYS_BIOCATALYSIS database, our tool offers high-accuracy predictions, reflecting the latest in chemical research and data.
Strategy Settings
Precursor scoring | Relevance Heuristic |
---|---|
Min. plausibility | 0.01 |
Model | Template_relevance |
Template Set | Pistachio/Bkms_metabolic/Pistachio_ringbreaker/Reaxys/Reaxys_biocatalysis |
Top-N result to add to graph | 6 |
Feasible Synthetic Routes
Disclaimer and Information on In-Vitro Research Products
Please be aware that all articles and product information presented on BenchChem are intended solely for informational purposes. The products available for purchase on BenchChem are specifically designed for in-vitro studies, which are conducted outside of living organisms. In-vitro studies, derived from the Latin term "in glass," involve experiments performed in controlled laboratory settings using cells or tissues. It is important to note that these products are not categorized as medicines or drugs, and they have not received approval from the FDA for the prevention, treatment, or cure of any medical condition, ailment, or disease. We must emphasize that any form of bodily introduction of these products into humans or animals is strictly prohibited by law. It is essential to adhere to these guidelines to ensure compliance with legal and ethical standards in research and experimentation.