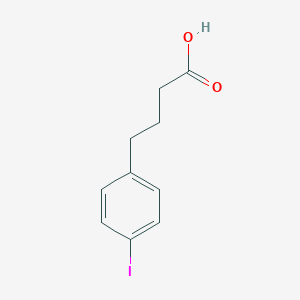
4-(p-Iodophenyl)butyric acid
Overview
Description
4-(p-Iodophenyl)butyric acid, also known as 4-iodobenzenebutanoic acid, is an organic compound with the molecular formula C10H11IO2 and a molecular weight of 290.10 g/mol . This compound is characterized by the presence of an iodine atom attached to a phenyl ring, which is further connected to a butyric acid moiety. It is a white to pale yellow solid that is sparingly soluble in chloroform and slightly soluble in methanol .
Mechanism of Action
Target of Action
4-(p-Iodophenyl)butyric acid is primarily used as a reactant in the synthesis of unsaturated amino acids, cyclophosphamide, and tetralone . It is also used as a portable albumin binder .
Mode of Action
The compound interacts with its targets through intramolecular Friedel-Crafts reactions . In the context of albumin binding, it binds non-covalently to naturally present albumin, which allows it to directly interact with tumor cells, promoting its cellular uptake .
Biochemical Pathways
The compound is involved in the synthesis of meta- and paracyclophanes containing unsaturated amino acids . It also participates in intramolecular Friedel-Crafts reactions for the synthesis of 1-tetralones .
Pharmacokinetics
It has been noted that when radioiodinated, it exhibits significantly improved pharmacokinetic properties . It is mainly accumulated in the blood with good retention .
Result of Action
The result of the compound’s action is the synthesis of unsaturated amino acids, cyclophosphamide, and tetralone . When used as an albumin binder, it extends the half-life of contrast agents .
Action Environment
The action of this compound can be influenced by environmental factors. For instance, its storage conditions are recommended to be 2-8°C . .
Preparation Methods
Synthetic Routes and Reaction Conditions
4-(p-Iodophenyl)butyric acid can be synthesized through several methods. One common approach involves the reaction of 4-iodonitrobenzene with propylene oxide to form 4-(4-iodophenyl)butyronitrile, which is then hydrolyzed to yield this compound . Another method involves the intramolecular Friedel-Crafts reaction of 4-iodobenzyl chloride with butyric acid in the presence of a Lewis acid catalyst .
Industrial Production Methods
Industrial production of this compound typically involves large-scale synthesis using the aforementioned methods. The reaction conditions are optimized to ensure high yield and purity. The product is then purified through recrystallization or chromatography techniques .
Chemical Reactions Analysis
Types of Reactions
4-(p-Iodophenyl)butyric acid undergoes various chemical reactions, including:
Substitution Reactions: The iodine atom can be substituted with other functional groups through nucleophilic substitution reactions.
Oxidation Reactions: The butyric acid moiety can be oxidized to form corresponding carboxylic acid derivatives.
Reduction Reactions: The phenyl ring can undergo reduction reactions to form cyclohexyl derivatives.
Common Reagents and Conditions
Substitution Reactions: Common reagents include sodium iodide and copper(I) iodide in the presence of a suitable solvent.
Oxidation Reactions: Reagents such as potassium permanganate or chromium trioxide are used under acidic conditions.
Reduction Reactions: Hydrogen gas in the presence of a palladium catalyst is commonly used[][7].
Major Products Formed
Substitution Reactions: Products include various substituted phenylbutyric acids.
Oxidation Reactions: Products include carboxylic acid derivatives.
Reduction Reactions: Products include cyclohexylbutyric acids.
Scientific Research Applications
4-(p-Iodophenyl)butyric acid has several scientific research applications:
Comparison with Similar Compounds
Similar Compounds
- 4-Phenylbutyric acid
- 4-Bromophenylbutyric acid
- 4-Chlorophenylbutyric acid
Comparison
4-(p-Iodophenyl)butyric acid is unique due to the presence of the iodine atom, which imparts distinct chemical and physical properties. Compared to 4-phenylbutyric acid, the iodine atom increases the molecular weight and alters the compound’s reactivity. The presence of iodine also makes it suitable for radiolabeling applications, which is not possible with 4-phenylbutyric acid . Similarly, 4-bromophenylbutyric acid and 4-chlorophenylbutyric acid have different reactivities and applications due to the presence of bromine and chlorine atoms, respectively .
Properties
IUPAC Name |
4-(4-iodophenyl)butanoic acid | |
---|---|---|
Source | PubChem | |
URL | https://pubchem.ncbi.nlm.nih.gov | |
Description | Data deposited in or computed by PubChem | |
InChI |
InChI=1S/C10H11IO2/c11-9-6-4-8(5-7-9)2-1-3-10(12)13/h4-7H,1-3H2,(H,12,13) | |
Source | PubChem | |
URL | https://pubchem.ncbi.nlm.nih.gov | |
Description | Data deposited in or computed by PubChem | |
InChI Key |
OGOMLUBUDYFIOG-UHFFFAOYSA-N | |
Source | PubChem | |
URL | https://pubchem.ncbi.nlm.nih.gov | |
Description | Data deposited in or computed by PubChem | |
Canonical SMILES |
C1=CC(=CC=C1CCCC(=O)O)I | |
Source | PubChem | |
URL | https://pubchem.ncbi.nlm.nih.gov | |
Description | Data deposited in or computed by PubChem | |
Molecular Formula |
C10H11IO2 | |
Source | PubChem | |
URL | https://pubchem.ncbi.nlm.nih.gov | |
Description | Data deposited in or computed by PubChem | |
DSSTOX Substance ID |
DTXSID50405208 | |
Record name | 4-(p-Iodophenyl)butyric acid | |
Source | EPA DSSTox | |
URL | https://comptox.epa.gov/dashboard/DTXSID50405208 | |
Description | DSSTox provides a high quality public chemistry resource for supporting improved predictive toxicology. | |
Molecular Weight |
290.10 g/mol | |
Source | PubChem | |
URL | https://pubchem.ncbi.nlm.nih.gov | |
Description | Data deposited in or computed by PubChem | |
CAS No. |
27913-58-2 | |
Record name | 4-(p-Iodophenyl)butyric acid | |
Source | EPA DSSTox | |
URL | https://comptox.epa.gov/dashboard/DTXSID50405208 | |
Description | DSSTox provides a high quality public chemistry resource for supporting improved predictive toxicology. | |
Synthesis routes and methods
Procedure details
Retrosynthesis Analysis
AI-Powered Synthesis Planning: Our tool employs the Template_relevance Pistachio, Template_relevance Bkms_metabolic, Template_relevance Pistachio_ringbreaker, Template_relevance Reaxys, Template_relevance Reaxys_biocatalysis model, leveraging a vast database of chemical reactions to predict feasible synthetic routes.
One-Step Synthesis Focus: Specifically designed for one-step synthesis, it provides concise and direct routes for your target compounds, streamlining the synthesis process.
Accurate Predictions: Utilizing the extensive PISTACHIO, BKMS_METABOLIC, PISTACHIO_RINGBREAKER, REAXYS, REAXYS_BIOCATALYSIS database, our tool offers high-accuracy predictions, reflecting the latest in chemical research and data.
Strategy Settings
Precursor scoring | Relevance Heuristic |
---|---|
Min. plausibility | 0.01 |
Model | Template_relevance |
Template Set | Pistachio/Bkms_metabolic/Pistachio_ringbreaker/Reaxys/Reaxys_biocatalysis |
Top-N result to add to graph | 6 |
Feasible Synthetic Routes
Q1: How does IPBA enhance the pharmacokinetic properties of radiopharmaceuticals?
A: IPBA acts as a non-covalent albumin binder. When conjugated to a radiopharmaceutical, it allows the drug to reversibly bind to albumin in the bloodstream [, , , , ]. This interaction increases the size of the drug complex, preventing rapid renal clearance and prolonging its circulation time [, , ]. Consequently, the drug has more opportunities to reach its target site, resulting in improved tumor uptake and retention [, , , ].
Q2: What is the impact of linker length and composition on the efficacy of IPBA-conjugated radiopharmaceuticals?
A: Research suggests that both linker length and composition play crucial roles in optimizing the tissue distribution profile of IPBA-conjugated radiopharmaceuticals [, ]. A study comparing different IPBA-modified PSMA ligands revealed that agents with longer linkers displayed significantly higher tumor uptake and retention compared to their shorter counterparts []. Additionally, varying the linker composition can influence the balance between tumor uptake and renal clearance. For instance, a long polyethylene glycol (PEG) linker resulted in the lowest blood and kidney accumulation while maintaining high tumor uptake []. This highlights the importance of carefully tailoring the linker structure to achieve an optimal pharmacokinetic profile.
Q3: Can you provide examples of specific radiopharmaceuticals that have been successfully modified with IPBA?
A3: Several studies showcase the successful application of IPBA in modifying radiopharmaceuticals targeting various biological systems. Some notable examples include:
- Melanoma: IPBA conjugation significantly enhanced the therapeutic efficacy of [177Lu]Lu-labeled-LLP2A, a radiopharmaceutical targeting very late antigen-4 (VLA-4) overexpressed in aggressive melanoma [].
- Prostate Cancer: A series of IPBA-modified PSMA-based low-molecular-weight radiotherapeutics demonstrated improved tumor targeting and retention compared to their non-modified counterparts [].
- Estrogen Receptor (ER) Positive Breast Cancer: An IPBA-modified estradiol derivative ([131I]IPBA-EE) showed promising results for ER targeting in SPECT imaging studies [].
- Fibroblast Activation Protein (FAP) Positive Tumors: Albumin binder–conjugated FAP inhibitors (including IPBA modifications) demonstrated significantly improved tumor uptake and retention, leading to remarkable growth inhibition of pancreatic cancer patient-derived xenograft (PDX) tumors [].
Q4: What are the potential advantages of using IPBA compared to other albumin-binding moieties?
A: While IPBA is a widely studied albumin binder, other moieties like Evans blue derivatives are also being explored for similar applications [, ]. A direct comparison between IPBA and a truncated Evans blue moiety conjugated to FAP inhibitors revealed that both modifications achieved prolonged blood circulation and improved tumor uptake []. This suggests that the choice of the optimal albumin binder may depend on the specific targeting molecule and desired pharmacokinetic profile. Further research is needed to fully elucidate the advantages and disadvantages of each albumin binder in different contexts.
Q5: Are there any safety concerns associated with using IPBA in radiopharmaceuticals?
A: While IPBA conjugation shows promise for improving radiopharmaceutical efficacy, its safety profile requires further investigation. The long-term effects of IPBA accumulation, particularly in the kidneys, warrant careful evaluation. Preclinical studies are crucial for assessing potential toxicity and determining the optimal dosing strategies to minimize adverse effects while maximizing therapeutic benefits [, , ].
Disclaimer and Information on In-Vitro Research Products
Please be aware that all articles and product information presented on BenchChem are intended solely for informational purposes. The products available for purchase on BenchChem are specifically designed for in-vitro studies, which are conducted outside of living organisms. In-vitro studies, derived from the Latin term "in glass," involve experiments performed in controlled laboratory settings using cells or tissues. It is important to note that these products are not categorized as medicines or drugs, and they have not received approval from the FDA for the prevention, treatment, or cure of any medical condition, ailment, or disease. We must emphasize that any form of bodily introduction of these products into humans or animals is strictly prohibited by law. It is essential to adhere to these guidelines to ensure compliance with legal and ethical standards in research and experimentation.