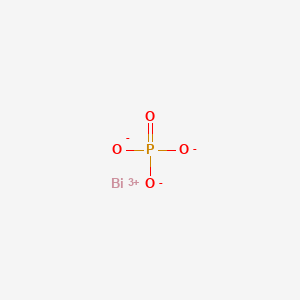
Bismuth phosphate
Overview
Description
Bismuth phosphate is an inorganic compound with the chemical formula BiPO₄. It is known for its unique properties, including high photocatalytic activity and photoluminescence. This compound is used in various applications, ranging from environmental engineering to biomedical fields. Its ability to degrade organic pollutants under UV light makes it a valuable material in photocatalysis .
Scientific Research Applications
Bismuth phosphate has a wide range of scientific research applications:
Safety and Hazards
Future Directions
Bismuth Phosphate has potential applications in environmental governance due to its small band gap and strong visible light response . Current research is focused on improving the photocatalytic performance of this compound for water treatment . There is also interest in exploring the biomedical applications of this compound .
Mechanism of Action
Target of Action
Bismuth phosphate, like other bismuth compounds, primarily targets the gastrointestinal tract . It has been used to treat ulcers caused by Helicobacter pylori . In addition, recent studies have shown that bismuth-based antivirals target the helicase of SARS-CoV-2 .
Mode of Action
This compound interacts with its targets through a complex molecular multi-targeted mode of action . Once inside the cells of H. pylori, bismuth may bind to various proteins/enzymes and in turn perturb a variety of biological pathways . In the case of SARS-CoV-2, bismuth-based antivirals inhibit the helicase, a critical protein in virus replication .
Biochemical Pathways
It is known that bismuth-based drugs can disrupt a variety of biological pathways once they bind to various proteins/enzymes within the target cells .
Pharmacokinetics
This is an area of ongoing research, with a focus on developing new bismuth compounds and approaches to overcome this challenge .
Result of Action
The result of this compound’s action is the alleviation of symptoms associated with the conditions it is used to treat. For instance, in the case of H. pylori infections, this compound can help heal ulcers and alleviate gastrointestinal discomfort . When used as an antiviral against SARS-CoV-2, it inhibits a critical protein in virus replication, thereby helping to control the infection .
Action Environment
The action of this compound can be influenced by various environmental factors. For instance, its photocatalytic properties can be utilized for environmental governance due to its small band gap and strong visible light response . .
Biochemical Analysis
Biochemical Properties
Bismuth phosphate interacts with various biomolecules in biochemical reactions. It has been found to generate surface active oxygen species that can react with other molecules
Cellular Effects
The effects of this compound on cells and cellular processes are complex and multifaceted. It has been suggested that it influences cell function, potentially impacting cell signaling pathways, gene expression, and cellular metabolism
Molecular Mechanism
It is believed to exert its effects at the molecular level through binding interactions with biomolecules, potential enzyme inhibition or activation, and changes in gene expression
Preparation Methods
Synthetic Routes and Reaction Conditions: Bismuth phosphate can be synthesized using several methods, including co-precipitation, solid-state reactions, and hydrothermal methods.
Co-precipitation Method: This involves mixing bismuth nitrate pentahydrate with a soluble phosphate source in an aqueous solution.
Solid-State Reaction: In this method, bismuth oxide and ammonium dihydrogen phosphate are mixed and heated at high temperatures (around 500°C) to form this compound.
Hydrothermal Method: This involves reacting bismuth nitrate with a phosphate source in a hydrothermal reactor at elevated temperatures (160°C) for several hours.
Industrial Production Methods: Industrial production of this compound typically involves large-scale co-precipitation or hydrothermal methods due to their simplicity and cost-effectiveness. The choice of method depends on the desired properties of the final product, such as particle size and crystallinity.
Chemical Reactions Analysis
Bismuth phosphate undergoes various chemical reactions, including:
Reduction: It can also participate in reduction reactions, although these are less common compared to oxidation.
Substitution: this compound can undergo substitution reactions where phosphate ions are replaced by other anions, altering its properties.
Common Reagents and Conditions:
Oxidation Reactions: Typically involve UV light and organic pollutants such as Rhodamine B or methylene blue.
Reduction Reactions: May involve reducing agents like hydrogen gas or hydrazine.
Substitution Reactions: Involve other anions such as sulfate or nitrate in aqueous solutions.
Major Products Formed:
Oxidation: Degradation products of organic pollutants, such as carbon dioxide and water.
Reduction: Reduced forms of this compound or other compounds.
Substitution: New bismuth compounds with different anions.
Comparison with Similar Compounds
Bismuth phosphate can be compared with other similar compounds, such as:
Bismuth Oxyhalides (BiOX): These compounds, where X can be chlorine, bromine, or iodine, also exhibit photocatalytic properties but have different band gaps and photocatalytic efficiencies.
Bismuth Oxide (Bi₂O₃): Known for its high refractive index and dielectric properties, bismuth oxide is used in optical coatings and electronic devices.
Bismuth Sulfide (Bi₂S₃): This compound is used in thermoelectric materials and has different electronic properties compared to this compound.
Uniqueness of this compound:
By understanding the unique properties and applications of this compound, researchers can continue to explore its potential in various scientific and industrial fields.
Properties
IUPAC Name |
bismuth;phosphate | |
---|---|---|
Source | PubChem | |
URL | https://pubchem.ncbi.nlm.nih.gov | |
Description | Data deposited in or computed by PubChem | |
InChI |
InChI=1S/Bi.H3O4P/c;1-5(2,3)4/h;(H3,1,2,3,4)/q+3;/p-3 | |
Source | PubChem | |
URL | https://pubchem.ncbi.nlm.nih.gov | |
Description | Data deposited in or computed by PubChem | |
InChI Key |
SFOQXWSZZPWNCL-UHFFFAOYSA-K | |
Source | PubChem | |
URL | https://pubchem.ncbi.nlm.nih.gov | |
Description | Data deposited in or computed by PubChem | |
Canonical SMILES |
[O-]P(=O)([O-])[O-].[Bi+3] | |
Source | PubChem | |
URL | https://pubchem.ncbi.nlm.nih.gov | |
Description | Data deposited in or computed by PubChem | |
Molecular Formula |
BiPO4, BiO4P | |
Record name | bismuth(III) orthophosphate | |
Source | Wikipedia | |
URL | https://en.wikipedia.org/wiki/Dictionary_of_chemical_formulas | |
Description | Chemical information link to Wikipedia. | |
Source | PubChem | |
URL | https://pubchem.ncbi.nlm.nih.gov | |
Description | Data deposited in or computed by PubChem | |
DSSTOX Substance ID |
DTXSID00884443 | |
Record name | Phosphoric acid, bismuth(3+) salt (1:1) | |
Source | EPA DSSTox | |
URL | https://comptox.epa.gov/dashboard/DTXSID00884443 | |
Description | DSSTox provides a high quality public chemistry resource for supporting improved predictive toxicology. | |
Molecular Weight |
303.952 g/mol | |
Source | PubChem | |
URL | https://pubchem.ncbi.nlm.nih.gov | |
Description | Data deposited in or computed by PubChem | |
Physical Description |
Odorless solid; Does not melt when heated; [Merck Index] | |
Record name | Bismuth phosphate | |
Source | Haz-Map, Information on Hazardous Chemicals and Occupational Diseases | |
URL | https://haz-map.com/Agents/12384 | |
Description | Haz-Map® is an occupational health database designed for health and safety professionals and for consumers seeking information about the adverse effects of workplace exposures to chemical and biological agents. | |
Explanation | Copyright (c) 2022 Haz-Map(R). All rights reserved. Unless otherwise indicated, all materials from Haz-Map are copyrighted by Haz-Map(R). No part of these materials, either text or image may be used for any purpose other than for personal use. Therefore, reproduction, modification, storage in a retrieval system or retransmission, in any form or by any means, electronic, mechanical or otherwise, for reasons other than personal use, is strictly prohibited without prior written permission. | |
CAS No. |
10049-01-1 | |
Record name | Bismuth phosphate | |
Source | ChemIDplus | |
URL | https://pubchem.ncbi.nlm.nih.gov/substance/?source=chemidplus&sourceid=0010049011 | |
Description | ChemIDplus is a free, web search system that provides access to the structure and nomenclature authority files used for the identification of chemical substances cited in National Library of Medicine (NLM) databases, including the TOXNET system. | |
Record name | Phosphoric acid, bismuth(3+) salt (1:1) | |
Source | EPA Chemicals under the TSCA | |
URL | https://www.epa.gov/chemicals-under-tsca | |
Description | EPA Chemicals under the Toxic Substances Control Act (TSCA) collection contains information on chemicals and their regulations under TSCA, including non-confidential content from the TSCA Chemical Substance Inventory and Chemical Data Reporting. | |
Record name | Phosphoric acid, bismuth(3+) salt (1:1) | |
Source | EPA DSSTox | |
URL | https://comptox.epa.gov/dashboard/DTXSID00884443 | |
Description | DSSTox provides a high quality public chemistry resource for supporting improved predictive toxicology. | |
Record name | Bismuth orthophosphate | |
Source | European Chemicals Agency (ECHA) | |
URL | https://echa.europa.eu/substance-information/-/substanceinfo/100.030.134 | |
Description | The European Chemicals Agency (ECHA) is an agency of the European Union which is the driving force among regulatory authorities in implementing the EU's groundbreaking chemicals legislation for the benefit of human health and the environment as well as for innovation and competitiveness. | |
Explanation | Use of the information, documents and data from the ECHA website is subject to the terms and conditions of this Legal Notice, and subject to other binding limitations provided for under applicable law, the information, documents and data made available on the ECHA website may be reproduced, distributed and/or used, totally or in part, for non-commercial purposes provided that ECHA is acknowledged as the source: "Source: European Chemicals Agency, http://echa.europa.eu/". Such acknowledgement must be included in each copy of the material. ECHA permits and encourages organisations and individuals to create links to the ECHA website under the following cumulative conditions: Links can only be made to webpages that provide a link to the Legal Notice page. | |
Record name | BISMUTH PHOSPHATE | |
Source | FDA Global Substance Registration System (GSRS) | |
URL | https://gsrs.ncats.nih.gov/ginas/app/beta/substances/Z9P58L0522 | |
Description | The FDA Global Substance Registration System (GSRS) enables the efficient and accurate exchange of information on what substances are in regulated products. Instead of relying on names, which vary across regulatory domains, countries, and regions, the GSRS knowledge base makes it possible for substances to be defined by standardized, scientific descriptions. | |
Explanation | Unless otherwise noted, the contents of the FDA website (www.fda.gov), both text and graphics, are not copyrighted. They are in the public domain and may be republished, reprinted and otherwise used freely by anyone without the need to obtain permission from FDA. Credit to the U.S. Food and Drug Administration as the source is appreciated but not required. | |
Retrosynthesis Analysis
AI-Powered Synthesis Planning: Our tool employs the Template_relevance Pistachio, Template_relevance Bkms_metabolic, Template_relevance Pistachio_ringbreaker, Template_relevance Reaxys, Template_relevance Reaxys_biocatalysis model, leveraging a vast database of chemical reactions to predict feasible synthetic routes.
One-Step Synthesis Focus: Specifically designed for one-step synthesis, it provides concise and direct routes for your target compounds, streamlining the synthesis process.
Accurate Predictions: Utilizing the extensive PISTACHIO, BKMS_METABOLIC, PISTACHIO_RINGBREAKER, REAXYS, REAXYS_BIOCATALYSIS database, our tool offers high-accuracy predictions, reflecting the latest in chemical research and data.
Strategy Settings
Precursor scoring | Relevance Heuristic |
---|---|
Min. plausibility | 0.01 |
Model | Template_relevance |
Template Set | Pistachio/Bkms_metabolic/Pistachio_ringbreaker/Reaxys/Reaxys_biocatalysis |
Top-N result to add to graph | 6 |
Feasible Synthetic Routes
Q1: What is the molecular formula and weight of bismuth phosphate?
A1: this compound, with the chemical formula BiPO4 [], has a molecular weight of 303.952 g/mol [].
Q2: What are the different polymorphic forms of this compound?
A2: this compound exists in three main polymorphic forms: a low-temperature monoclinic phase, a high-temperature monoclinic phase, and a hexagonal phase [, ]. The hexagonal phase can be slightly hydrated [].
Q3: What spectroscopic techniques are used to characterize this compound?
A3: Researchers commonly utilize a variety of spectroscopic methods to analyze this compound, including X-ray diffraction (XRD) [, , , ], Fourier transform infrared (FTIR) spectroscopy [, ], Raman spectroscopy [, , ], X-ray photoelectron spectroscopy (XPS) [], and 31P magic angle spinning nuclear magnetic resonance (MAS NMR) spectroscopy [].
Q4: How does the addition of this compound affect the properties of calcium phosphate cement (CPC)?
A4: Incorporating this compound into CPC as a radiopaque agent significantly improves radiopacity, even at low concentrations (9%) []. It also enhances injectability by reducing viscosity, and at concentrations up to 12%, improves compressive strength [].
Q5: How does doping with lanthanide ions (Ln3+) affect the thermal stability of this compound nanocrystals?
A5: Doping this compound nanocrystals with a small amount (≤9%) of Ln3+ ions, such as La3+, Ce3+, and Nd3+, remarkably enhances the thermal stability of the low-temperature monoclinic phase, preventing its complete transition to the high-temperature monoclinic phase even at high temperatures like 900 °C [].
Q6: What factors influence the formation of different this compound crystal structures?
A6: Synthesis conditions like temperature and pH significantly influence the resulting this compound polymorph. Lower temperatures and higher acidity generally favor the formation of the trigonal phase [].
Q7: How does this compound perform as a photocatalyst?
A7: this compound exhibits promising photocatalytic activity, effectively degrading pollutants like methylene blue and methylene orange under UV-visible light irradiation [, ]. The morphology and structure of the catalyst, influenced by synthesis parameters, can impact its photocatalytic efficiency [].
Q8: How does the presence of transition metal cations affect the catalytic activity of multicomponent this compound catalysts in propylene ammoxidation?
A8: The addition of transition metal cations, like molybdenum, to multicomponent this compound catalysts can significantly influence their catalytic activity and selectivity in reactions like propylene ammoxidation []. This modification highlights the potential of tailoring catalytic performance through compositional changes.
Q9: Can the reduction of this compound catalysts with propylene provide insights into their catalytic mechanisms?
A9: Studying the reduction of this compound catalysts with propylene reveals valuable information about their redox behavior during reactions like oxidative dehydroaromatization of olefins. The observed changes in CO2 and benzene formation during reduction suggest a redox mechanism involving Bi3+ and Bi [].
Q10: What are some of the potential applications of this compound?
A10: this compound holds potential for various applications, including:
- Radiopaque agent in CPC: Due to its ability to enhance radiopacity [].
- Photocatalyst: For environmental remediation due to its ability to degrade organic pollutants under UV-visible light [, ].
- Catalyst in organic synthesis: For reactions like propylene ammoxidation [] and oxidative dehydroaromatization of olefins [].
- Luminescent host material: When doped with rare-earth ions, it can exhibit tunable luminescence properties for potential applications in photonics [, ].
Q11: How is this compound used in the treatment of hydroceles?
A11: While this compound has been historically used as a sclerosant for hydroceles, its use has declined due to reported severe pain upon injection []. Other sclerosing agents with potentially fewer side effects are now preferred for this application.
Q12: What is the historical significance of this compound in nuclear fuel reprocessing?
A12: this compound played a crucial historical role in the separation of plutonium from irradiated nuclear fuel, specifically in the this compound Process [, ]. While no longer in use, understanding its behavior in this process remains important for managing legacy nuclear waste.
Q13: What factors influence the dissolution of this compound in caustic solutions?
A13: Caustic leaching studies on Hanford tank sludge, which contains this compound, reveal that factors such as hydroxide ion concentration, temperature, and the presence of other metal phosphates influence the rate and extent of this compound dissolution [].
Q14: How does the solubility of this compound impact its effectiveness in different applications?
A14: The solubility of this compound in various media plays a crucial role in determining its bioavailability and efficacy in different applications. For instance, in medical applications like sclerotherapy, its solubility can impact its diffusion and distribution within the target tissue [].
Q15: How is this compound quantified in different matrices?
A15: Researchers employ various analytical techniques to quantify this compound in different matrices. For instance, in the context of nuclear waste analysis, techniques like inductively coupled plasma mass spectrometry (ICP-MS) can be used to determine the concentration of bismuth, providing insights into the amount of this compound present [].
Q16: Are there any alternatives to this compound in its various applications?
A16: Yes, depending on the specific application, several alternatives to this compound exist. For example, other radiopaque agents like barium sulfate or tantalum oxide can be used in CPC instead of this compound []. Similarly, alternative photocatalytic materials, such as titanium dioxide (TiO2), are widely studied and employed for environmental remediation purposes [].
Q17: What resources are available to researchers studying this compound?
A17: Researchers exploring this compound have access to a wide range of resources, including:
Q18: What are some of the key historical milestones in the research and application of this compound?
A18: Key historical milestones related to this compound include:
Disclaimer and Information on In-Vitro Research Products
Please be aware that all articles and product information presented on BenchChem are intended solely for informational purposes. The products available for purchase on BenchChem are specifically designed for in-vitro studies, which are conducted outside of living organisms. In-vitro studies, derived from the Latin term "in glass," involve experiments performed in controlled laboratory settings using cells or tissues. It is important to note that these products are not categorized as medicines or drugs, and they have not received approval from the FDA for the prevention, treatment, or cure of any medical condition, ailment, or disease. We must emphasize that any form of bodily introduction of these products into humans or animals is strictly prohibited by law. It is essential to adhere to these guidelines to ensure compliance with legal and ethical standards in research and experimentation.