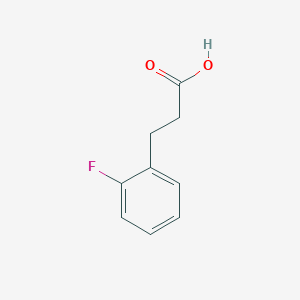
3-(2-Fluorophenyl)propionic acid
Overview
Description
3-(2-Fluorophenyl)propionic acid is a fluorinated aromatic propionic acid derivative characterized by a fluorine atom at the ortho position of the phenyl ring attached to a three-carbon propionic acid chain. Its synthesis involves the condensation of 2-fluorobenzaldehyde with malonic acid and ammonium acetate under reflux in ethanol, yielding the compound with a moderate efficiency (58.6%) . This compound serves as a key intermediate in medicinal chemistry, particularly in synthesizing dihydroquinazoline-2(1H)-one derivatives for drug discovery and phosphonic acid analogues targeting alanyl aminopeptidases . Its structural features, including the electron-withdrawing fluorine substituent and carboxylic acid group, influence its physicochemical and biological properties, making it distinct from non-fluorinated or differently substituted analogs.
Preparation Methods
Malonic Ester Synthesis via Alkylation and Hydrolysis
The malonic ester synthesis remains the most widely adopted method for producing 3-(2-fluorophenyl)propionic acid due to its modularity and high yields . This two-step process involves:
Alkylation of Dimethyl Malonate
In a nitrogen-purged reactor, sodium methoxide (0.26 mol) is dissolved in anhydrous methanol (100 mL) at 40°C. Dimethyl malonate (1.0 mol) is added dropwise, followed by 2-fluorobenzyl chloride (0.2 mol). The mixture is refluxed for 2–3 hours, yielding 2-(2-fluorobenzyl)dimethyl malonate as a pale-yellow oil. Key parameters:
-
Solvent : Methanol (polar, protic) enhances nucleophilicity of malonate enolate.
-
Temperature : 40–50°C minimizes side reactions (e.g., ester hydrolysis).
-
Yield : 92–95% after vacuum distillation to recover unreacted dimethyl malonate .
Acid-Catalyzed Hydrolysis and Decarboxylation
The alkylated malonate ester (0.19 mol) is hydrolyzed using 37% hydrochloric acid (73 mL) under reflux for 5 hours. The reaction proceeds via:
-
Ester hydrolysis to 2-(2-fluorobenzyl)malonic acid.
-
Decarboxylation at 100–110°C to form this compound.
Optimization Insights :
-
Acid Concentration : ≥30% HCl ensures complete decarboxylation.
-
Reaction Time : Prolonged reflux (>5 hours) reduces yield due to thermal decomposition.
-
Isolation : Cooling the reaction mixture to 10°C precipitates the product, which is filtered and recrystallized from acetonitrile (purity >98%) .
Table 1: Performance Metrics for Malonic Ester Route
Parameter | Value |
---|---|
Overall Yield | 85–89% |
Purity (HPLC) | 97–99% |
Reaction Scale (Industrial) | 50–100 kg/batch |
Energy Consumption | 15 kWh/kg |
Hydrolysis of Nitrile Precursors
An alternative route involves the hydrolysis of 3-(2-fluorophenyl)propionitrile, though this method is less common due to handling challenges with cyanide intermediates.
Synthesis of 3-(2-Fluorophenyl)propionitrile
2-Fluorobenzyl chloride (1.0 eq) is reacted with cyanoacetic acid (1.2 eq) in the presence of potassium carbonate (2.0 eq) in DMF at 80°C for 12 hours. The nitrile intermediate is isolated via extraction with ethyl acetate (yield: 78–82%).
Acidic Hydrolysis to Propionic Acid
The nitrile is hydrolyzed using 6M sulfuric acid at 120°C for 8 hours. Excess acid is neutralized with NaOH, and the product is extracted into dichloromethane.
Challenges :
-
Toxicity : Requires stringent controls for cyanide exposure.
-
Byproducts : Partial oxidation to amides may occur, necessitating chromatographic purification.
Table 2: Nitrile Hydrolysis vs. Malonic Ester Route
Metric | Nitrile Hydrolysis | Malonic Ester Synthesis |
---|---|---|
Yield | 70–75% | 85–89% |
Purity | 90–93% | 97–99% |
Scalability | Limited to 10 kg/batch | >50 kg/batch |
Cyanide Waste Generated | 0.5 kg/kg product | None |
Grignard Reaction with Subsequent Oxidation
This three-step method is favored for laboratory-scale synthesis but is cost-prohibitive for industrial applications.
Formation of 2-Fluorophenylmagnesium Bromide
Magnesium turnings (1.1 eq) are activated with iodine in dry THF. 2-Fluorobromobenzene (1.0 eq) is added dropwise under reflux to form the Grignard reagent.
Reaction with Acrylic Acid Derivatives
The Grignard reagent is quenched with methyl acrylate (1.0 eq) at −10°C, yielding methyl 3-(2-fluorophenyl)propionate after workup (yield: 65–70%).
Saponification to Propionic Acid
The ester is hydrolyzed with 2M NaOH in ethanol/water (1:1) under reflux for 3 hours. Acidification with HCl precipitates the product (purity: 95%).
Drawbacks :
-
Moisture Sensitivity : Grignard reagents require anhydrous conditions.
-
Cost : THF and magnesium increase raw material expenses.
Method | Yield | Purity | Scalability | Enantiocontrol |
---|---|---|---|---|
Malonic Ester | 85–89% | 97–99% | High | None |
Nitrile Hydrolysis | 70–75% | 90–93% | Moderate | None |
Grignard/Oxidation | 65–70% | 95% | Low | None |
Biocatalytic | 40–45% | 85–90% | Pilot-scale | Yes (ee >99%) |
Critical Process Optimization Strategies
Solvent Selection for Alkylation
-
Polar Aprotic Solvents (DMF, DMSO) : Increase reaction rate but complicate purification.
-
Ethers (THF) : Provide moderate polarity with easier solvent recovery.
-
Methanol : Optimal balance between enolate formation and cost .
Catalytic Enhancements
-
Phase-Transfer Catalysts : Tetrabutylammonium bromide (0.1 eq) improves alkylation yield by 8–10%.
-
Microwave Assistance : Reduces malonic ester synthesis time from 5 hours to 45 minutes (80°C, 300 W).
Waste Minimization Techniques
-
Recycling Unreacted Malonate : Distillation recovers 80–85% of dimethyl malonate for reuse.
-
Neutralization Byproducts : Sodium chloride from HCl hydrolysis is converted to commercial-grade salt via crystallization.
Industrial-Scale Production Considerations
Equipment Specifications
-
Reactor Material : Glass-lined steel to resist HCl corrosion.
-
Distillation Columns : Packed columns with Hastelloy C-276 trays for malonate recovery.
Regulatory Compliance
-
EPA Guidelines : Cyanide levels in effluent <0.2 ppm (nitrile route).
-
OSHA Standards : Airborne fluoride exposure <2.5 mg/m³.
Chemical Reactions Analysis
Types of Reactions:
Oxidation: 3-(2-Fluorophenyl)propionic acid can undergo oxidation reactions to form corresponding carboxylic acids or ketones.
Reduction: It can be reduced to form alcohols or alkanes.
Substitution: The compound can participate in electrophilic aromatic substitution reactions due to the presence of the fluorophenyl group.
Common Reagents and Conditions:
Oxidation: Common oxidizing agents include potassium permanganate and chromium trioxide.
Reduction: Reducing agents such as lithium aluminum hydride or sodium borohydride are often used.
Substitution: Reagents like halogens (chlorine, bromine) and nitrating agents can be used under controlled conditions.
Major Products:
Oxidation: Formation of 3-(2-Fluorophenyl)propanoic acid.
Reduction: Formation of 3-(2-Fluorophenyl)propanol.
Substitution: Formation of various substituted fluorophenyl derivatives.
Scientific Research Applications
Pharmaceutical Development
3-(2-Fluorophenyl)propionic acid serves as a building block in the synthesis of pharmaceutical agents. Its structural properties make it valuable for designing drugs that target neurological disorders. The presence of the fluorine atom enhances the lipophilicity of compounds, improving their ability to cross biological membranes and interact with cellular targets.
Key Insights:
- Modulator of Neurotransmitter Systems: Research indicates that this compound can modulate neurotransmitter systems, paving the way for innovative treatments for conditions such as depression and anxiety .
- Drug Design: The unique electronic properties imparted by fluorine allow researchers to design novel compounds with improved efficacy and reduced side effects .
Neuroscience Research
In neuroscience, this compound is utilized to study synaptic transmission and neurotransmitter interactions. Its role as an amino acid analog enables researchers to investigate the effects of amino acid modifications on protein function.
Research Applications:
- Biochemical Assays: Employed in assays to evaluate protein interactions, aiding in understanding the mechanisms underlying neurotransmission .
- Potential Treatments: Studies have explored its potential in developing therapeutic strategies for neurological diseases by influencing synaptic activity .
Biochemical Assays
The compound is extensively used in biochemical assays to assess the effects of amino acid modifications on protein function. This application is crucial for understanding how alterations in amino acid structures can impact biological processes.
Applications:
- Protein Interaction Studies: Helps elucidate how changes in amino acid composition affect protein interactions and functions .
- Mechanistic Studies: Provides insights into enzyme mechanisms and metabolic pathways by serving as a substrate or inhibitor .
Analytical Chemistry
In analytical chemistry, this compound is used as a standard for calibrating instruments and ensuring accurate measurements in various chemical analyses.
Significance:
- Calibration Standards: Acts as a reference compound in chromatographic methods such as HPLC and LC-MS, facilitating accurate quantification of other compounds .
- Chemical Reactions Analysis: Its reactivity allows for the study of various chemical reactions, including oxidation and reduction processes.
The biological activity of this compound has been investigated for its potential antimicrobial and anticancer properties.
Case Studies:
- Antimicrobial Activity: Preliminary studies indicate its effectiveness against several bacterial strains due to its ability to disrupt microbial membranes.
- Anticancer Properties: Investigations have shown that it may inhibit cancer cell proliferation through apoptosis induction, activating caspases involved in cell death pathways.
Mechanism of Action
The mechanism by which 3-(2-Fluorophenyl)propionic acid exerts its effects involves interactions with specific molecular targets and pathways. The fluorophenyl group can interact with various enzymes and receptors, influencing biological processes. The exact pathways and targets can vary depending on the specific application and context of use .
Comparison with Similar Compounds
Comparison with Structurally Similar Compounds
Substituent Position: Ortho vs. Para Fluorine
- 3-(4-Fluorophenyl)propionic Acid: The para-fluorine isomer (CAS 459-31-4) shares a similar molecular weight (168.167 g/mol) and formula (C₉H₉FO₂) with the ortho-fluoro derivative . This positional difference may alter solubility and bioavailability, though direct comparative data are lacking.
- Reactivity : The ortho-fluorine in 3-(2-fluorophenyl)propionic acid introduces greater steric and electronic effects compared to the para isomer, which could influence its reactivity in esterification or amidation reactions .
Hydroxylated Analogs: Antioxidant and Metabolic Profiles
- The hydroxyl group at the para position enhances radical scavenging via hydrogen donation, a mechanism less accessible to the fluorine-substituted analog.
- Microbial Metabolism: Hydroxylated derivatives like 3-(3,4-dihydroxyphenyl)propionic acid are rapidly metabolized by gut microbiota, peaking within 2–6 hours, whereas non-hydroxylated analogs (e.g., phenylpropionic acid) accumulate over 20 hours . Fluorine’s electron-withdrawing nature may slow enzymatic degradation, extending half-life in biological systems.
Methoxy-Substituted Derivatives: Anti-inflammatory Potential
- 3-(p-Methoxyphenyl)propionic Acid: This compound interacts with TNF-α and IL-1β receptors, suggesting anti-inflammatory properties comparable to metformin .
Structural and Functional Data Table
Key Research Findings
- Antioxidant Activity : Hydroxyl groups significantly enhance radical scavenging, while fluorine’s electronegativity may reduce electron donation capacity .
- Metabolic Stability : Fluorinated derivatives likely resist microbial degradation better than hydroxylated analogs, prolonging their presence in biological systems .
- Synthetic Utility : The ortho-fluoro substituent in this compound facilitates steric control in multi-step syntheses, such as dihydroquinazoline scaffolds .
Biological Activity
3-(2-Fluorophenyl)propionic acid (CAS Number: 1643-26-1) is a compound of interest due to its potential biological activities and applications in medicinal chemistry. This article summarizes the biological activity of this compound, including its synthesis, mechanisms of action, and relevant case studies.
- Molecular Formula : C₉H₉FO₂
- Molecular Weight : 168.17 g/mol
- Melting Point : 76-78 °C
- Hazard Classification : Irritant
Synthesis
The synthesis of this compound can be achieved through various methods, including enzymatic routes and chemical processes. One notable method involves the use of phenylalanine aminomutase (PAM), which catalyzes the conversion of substituted cinnamic acids into enantiopure amino acids .
-
Inhibition of Histone Deacetylases (HDACs) :
- Recent studies indicate that compounds related to this compound exhibit inhibitory activity against HDACs, which are crucial in regulating gene expression and cellular functions. The pIC₅₀ values for various polypeptide compounds derived from similar structures suggest significant HDAC inhibition, with values ranging from 6.04 to 7.23 .
- Antioxidant Properties :
-
Interaction with Neurotransmitter Systems :
- The presence of fluorine in the phenyl ring can enhance the lipophilicity and bioavailability of the compound, potentially affecting neurotransmitter interactions and leading to psychotropic effects.
Case Studies
- Neuroprotective Effects :
- Anticancer Potential :
Table 1: Inhibitory Activity of Related Compounds on HDACs
Compound Name | pIC₅₀ Value |
---|---|
Compound 1 | 7.23 |
Compound 2 | 6.85 |
Compound 3 | 6.27 |
Compound 4 | 6.92 |
Compound 5 | 6.08 |
Compound 6 | 6.12 |
Compound 7 | 6.04 |
Q & A
Q. Basic: What are the standard synthetic routes for 3-(2-fluorophenyl)propionic acid, and how can reaction conditions be optimized for yield?
Methodological Answer:
A common synthesis involves the condensation of 2-fluorobenzaldehyde with malonic acid in ethanol under reflux, using ammonium acetate as a catalyst. For example, refluxing for 12–24 hours yields 3-amino-3-(2-fluorophenyl)propionic acid with ~58% efficiency . Optimization strategies include:
- Solvent selection : Ethanol or acetonitrile improves solubility and reduces side reactions.
- Catalyst loading : Adjusting ammonium acetate (e.g., 0.5–1.0 mmol) balances reaction rate and byproduct formation.
- Temperature control : Prolonged reflux (~80°C) enhances cyclization but may require inert atmospheres to prevent oxidation.
Post-synthesis, recrystallization in acetonitrile or cold ethanol yields pure crystals suitable for X-ray analysis .
Q. Advanced: How do crystallographic studies resolve molecular conformation and intramolecular interactions in this compound derivatives?
Methodological Answer:
X-ray crystallography (e.g., using SHELXL ) reveals key structural features:
- Bond length anomalies : The C=O bond in the carboxyl group is shorter (1.306 Å vs. 1.325 Å in analogues) due to electron delocalization .
- Conformational analysis : Derivatives adopt anti-conformations (torsion angle: 173.8°) rather than gauche (64.9°), influenced by steric hindrance from the 2-fluorophenyl group .
- Hydrogen bonding : Intramolecular N–H···F and N–H···O bonds form pseudo-six-membered rings, stabilizing the planar thiourea and carboxyl fragments (dihedral angles: 20.84° and 85.78° with fluorophenyl/propionic acid groups) .
Refinement tips : Use isotropic refinement for hydroxyl H-atoms and riding models for others (C–H = 0.93–0.97 Å) .
Q. Basic: Which spectroscopic techniques are most effective for characterizing this compound?
Methodological Answer:
- Mass spectrometry (LC-ESI-QQ) : Identifies the [M–H]⁻ ion at m/z 182.08 (calculated for C₉H₈FO₂⁻) and fragments (e.g., loss of CO₂ at m/z 138) .
- FT-IR : Key peaks include ν(C=O) at ~1700 cm⁻¹ and ν(C–F) at 1100–1250 cm⁻¹.
- ¹H/¹³C NMR : Look for aromatic protons (δ 7.2–7.8 ppm, split by ortho-F coupling) and the propionic acid chain (δ 2.6–3.1 ppm for CH₂) .
Q. Advanced: How can computational methods predict electronic properties and reactivity of this compound?
Methodological Answer:
Density Functional Theory (DFT) calculations (e.g., B3LYP/6-311+G(d,p)) provide insights:
- Electrostatic potential maps : Highlight nucleophilic regions (carboxyl group) and electrophilic sites (fluorophenyl ring) .
- Solubility parameters : Calculate δ (cohesive energy density) to predict compatibility with excipients (e.g., δ ≈ 22 MPa¹/² for polar solvents) .
- Reactivity indices : Fukui functions identify sites prone to electrophilic attack (e.g., para to fluorine) .
Validation : Compare computed vibrational spectra with experimental IR/Raman data to refine models.
Q. Basic: What are the common challenges in purifying this compound?
Methodological Answer:
- Byproduct formation : Unreacted 2-fluorobenzoyl chloride or thiourea adducts may persist. Use column chromatography (silica gel, ethyl acetate/hexane) for separation.
- Recrystallization : Acetonitrile is optimal for high-purity crystals; avoid protic solvents (e.g., water) to prevent hydrolysis .
- Yield loss : Cold washing with ethanol removes impurities without dissolving the product .
Q. Advanced: How should researchers reconcile discrepancies in bond lengths or conformations between structural analogues?
Methodological Answer:
- Comparative crystallography : Analyze datasets (e.g., CIF files) for analogous compounds (e.g., 3-(3-methoxyphenyl)propionic acid ) to identify trends.
- Electron density maps : Overlay structures to assess steric/electronic effects (e.g., fluorine’s electronegativity shortening C–O bonds) .
- Statistical validation : Use R-factors and goodness-of-fit (GoF) metrics to evaluate refinement quality across studies .
Q. Basic: What safety protocols are essential when handling this compound?
Methodological Answer:
- Ventilation : Use fume hoods to avoid inhalation of fluorinated vapors .
- PPE : Nitrile gloves and lab coats prevent dermal exposure; rinse immediately with water if contact occurs .
- Waste disposal : Neutralize acidic waste with bicarbonate before disposal .
Q. Advanced: How does fluorination at the 2-position influence the compound’s bioactivity in drug design?
Methodological Answer:
- Metabolic stability : Fluorine reduces oxidative metabolism, prolonging half-life .
- Target binding : The 2-fluoro group enhances π-stacking with aromatic residues (e.g., in kinase inhibitors) .
- Toxicity screening : Use in vitro assays (e.g., CYP450 inhibition) to assess fluorinated metabolite risks .
Properties
IUPAC Name |
3-(2-fluorophenyl)propanoic acid | |
---|---|---|
Source | PubChem | |
URL | https://pubchem.ncbi.nlm.nih.gov | |
Description | Data deposited in or computed by PubChem | |
InChI |
InChI=1S/C9H9FO2/c10-8-4-2-1-3-7(8)5-6-9(11)12/h1-4H,5-6H2,(H,11,12) | |
Source | PubChem | |
URL | https://pubchem.ncbi.nlm.nih.gov | |
Description | Data deposited in or computed by PubChem | |
InChI Key |
GUZLQEOSDXLCKX-UHFFFAOYSA-N | |
Source | PubChem | |
URL | https://pubchem.ncbi.nlm.nih.gov | |
Description | Data deposited in or computed by PubChem | |
Canonical SMILES |
C1=CC=C(C(=C1)CCC(=O)O)F | |
Source | PubChem | |
URL | https://pubchem.ncbi.nlm.nih.gov | |
Description | Data deposited in or computed by PubChem | |
Molecular Formula |
C9H9FO2 | |
Source | PubChem | |
URL | https://pubchem.ncbi.nlm.nih.gov | |
Description | Data deposited in or computed by PubChem | |
DSSTOX Substance ID |
DTXSID00366370 | |
Record name | 3-(2-Fluorophenyl)propionic acid | |
Source | EPA DSSTox | |
URL | https://comptox.epa.gov/dashboard/DTXSID00366370 | |
Description | DSSTox provides a high quality public chemistry resource for supporting improved predictive toxicology. | |
Molecular Weight |
168.16 g/mol | |
Source | PubChem | |
URL | https://pubchem.ncbi.nlm.nih.gov | |
Description | Data deposited in or computed by PubChem | |
CAS No. |
1643-26-1 | |
Record name | 3-(2-Fluorophenyl)propionic acid | |
Source | EPA DSSTox | |
URL | https://comptox.epa.gov/dashboard/DTXSID00366370 | |
Description | DSSTox provides a high quality public chemistry resource for supporting improved predictive toxicology. | |
Record name | 3-(2-fluorophenyl)propanoic acid | |
Source | European Chemicals Agency (ECHA) | |
URL | https://echa.europa.eu/information-on-chemicals | |
Description | The European Chemicals Agency (ECHA) is an agency of the European Union which is the driving force among regulatory authorities in implementing the EU's groundbreaking chemicals legislation for the benefit of human health and the environment as well as for innovation and competitiveness. | |
Explanation | Use of the information, documents and data from the ECHA website is subject to the terms and conditions of this Legal Notice, and subject to other binding limitations provided for under applicable law, the information, documents and data made available on the ECHA website may be reproduced, distributed and/or used, totally or in part, for non-commercial purposes provided that ECHA is acknowledged as the source: "Source: European Chemicals Agency, http://echa.europa.eu/". Such acknowledgement must be included in each copy of the material. ECHA permits and encourages organisations and individuals to create links to the ECHA website under the following cumulative conditions: Links can only be made to webpages that provide a link to the Legal Notice page. | |
Synthesis routes and methods I
Procedure details
Synthesis routes and methods II
Procedure details
Synthesis routes and methods III
Procedure details
Retrosynthesis Analysis
AI-Powered Synthesis Planning: Our tool employs the Template_relevance Pistachio, Template_relevance Bkms_metabolic, Template_relevance Pistachio_ringbreaker, Template_relevance Reaxys, Template_relevance Reaxys_biocatalysis model, leveraging a vast database of chemical reactions to predict feasible synthetic routes.
One-Step Synthesis Focus: Specifically designed for one-step synthesis, it provides concise and direct routes for your target compounds, streamlining the synthesis process.
Accurate Predictions: Utilizing the extensive PISTACHIO, BKMS_METABOLIC, PISTACHIO_RINGBREAKER, REAXYS, REAXYS_BIOCATALYSIS database, our tool offers high-accuracy predictions, reflecting the latest in chemical research and data.
Strategy Settings
Precursor scoring | Relevance Heuristic |
---|---|
Min. plausibility | 0.01 |
Model | Template_relevance |
Template Set | Pistachio/Bkms_metabolic/Pistachio_ringbreaker/Reaxys/Reaxys_biocatalysis |
Top-N result to add to graph | 6 |
Feasible Synthetic Routes
Disclaimer and Information on In-Vitro Research Products
Please be aware that all articles and product information presented on BenchChem are intended solely for informational purposes. The products available for purchase on BenchChem are specifically designed for in-vitro studies, which are conducted outside of living organisms. In-vitro studies, derived from the Latin term "in glass," involve experiments performed in controlled laboratory settings using cells or tissues. It is important to note that these products are not categorized as medicines or drugs, and they have not received approval from the FDA for the prevention, treatment, or cure of any medical condition, ailment, or disease. We must emphasize that any form of bodily introduction of these products into humans or animals is strictly prohibited by law. It is essential to adhere to these guidelines to ensure compliance with legal and ethical standards in research and experimentation.