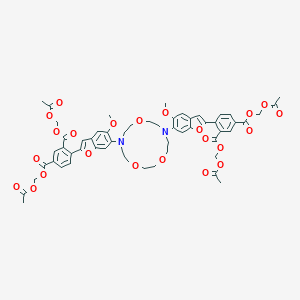
Sbfi-AM
Overview
Description
Sodium-binding benzofuran isophthalate acetoxymethyl ester (SBFI AM) is a fluorescent sodium indicator dye. It is widely used in biological and chemical research to measure intracellular sodium ion concentrations. This compound is particularly valuable due to its ability to selectively bind sodium ions over potassium ions, making it a useful tool for studying sodium dynamics in various cellular processes .
Mechanism of Action
Target of Action
Sbfi-AM, also known as Sodium-binding Benzofuran Isophthalate Acetoxymethyl ester, is a sodium-sensitive molecule . It primarily targets sodium ions (Na+) in cells . The role of these targets is crucial in various cellular functions, including the generation of action potentials and resting membrane potentials in excitable cells like neurons and cardiac muscle cells .
Mode of Action
This compound interacts with its targets (Na+ ions) by binding to them . This binding results in an increase in fluorescence intensity . The spectral response of this compound upon ion binding permits excitation ratio measurements , which can be used with the same optical filters and equipment used for other indicators like Fura-2 .
Biochemical Pathways
This compound is used to estimate Na+ gradients in isolated mitochondria, measure intracellular Na+ levels, and determine Na+ efflux in cells . It can also be used in combination with other fluorescent indicators to correlate changes in intracellular Na+ with Ca2+ and Mg2+ concentrations, intracellular pH, and membrane potential .
Pharmacokinetics
The pharmacokinetics of this compound involves its ability to permeate cell membranes . Once inside the cell, it binds to Na+ ions, which leads to changes in its fluorescence intensity . The dissociation constant (Kd) of this compound for Na+ is 3.8 mM in the absence of K+, and 11.3 mM in solutions with a combined Na+ and K+ concentration of 135 mM .
Result of Action
The binding of this compound to Na+ ions results in changes in the fluorescence intensity, which can be measured . This allows for the detection of physiological concentrations of Na+ in the presence of other monovalent cations . It also enables the correlation of changes in intracellular Na+ with other ions and cellular parameters .
Action Environment
The action of this compound can be influenced by environmental factors such as the presence of other ions. For instance, its selectivity for Na+ is about 18 times stronger than for K+ . Additionally, the presence of other fluorescent indicators can affect its ability to correlate changes in intracellular Na+ with other ions and cellular parameters .
Biochemical Analysis
Biochemical Properties
Sbfi-AM plays a significant role in biochemical reactions. It interacts with various enzymes, proteins, and other biomolecules. For instance, it is used to estimate Na+ gradients in isolated mitochondria, measure intracellular Na+ levels, and measure Na+ efflux in cells . Furthermore, it is used in combination with other fluorescent indicators to correlate changes in intracellular Na+ with Ca2+ and Mg2+ concentrations, intracellular pH, and membrane potential .
Cellular Effects
This compound has profound effects on various types of cells and cellular processes. It influences cell function by impacting cell signaling pathways, gene expression, and cellular metabolism . For example, it can be used to detect changes in the membrane potential of neurons and myocytes .
Molecular Mechanism
The mechanism of action of this compound operates at the molecular level. It exerts its effects through binding interactions with biomolecules, enzyme inhibition or activation, and changes in gene expression . For instance, it inhibits specific enzymes, such as tyrosine kinases, which help to regulate cell growth and differentiation .
Temporal Effects in Laboratory Settings
In laboratory settings, the effects of this compound change over time. It has been observed that the product’s stability, degradation, and long-term effects on cellular function vary in in vitro or in vivo studies .
Dosage Effects in Animal Models
The effects of this compound vary with different dosages in animal models. Studies have observed threshold effects, as well as toxic or adverse effects at high doses .
Metabolic Pathways
This compound is involved in various metabolic pathways. It interacts with enzymes or cofactors, and can affect metabolic flux or metabolite levels .
Transport and Distribution
This compound is transported and distributed within cells and tissues. It interacts with transporters or binding proteins, and can affect its localization or accumulation .
Subcellular Localization
The subcellular localization of this compound and its effects on its activity or function are significant. It can be directed to specific compartments or organelles based on targeting signals or post-translational modifications .
Preparation Methods
Synthetic Routes and Reaction Conditions
Sodium-binding benzofuran isophthalate acetoxymethyl ester is synthesized through a series of chemical reactions involving the coupling of benzofuran derivatives with isophthalate esters. The synthesis typically involves the following steps:
Formation of Benzofuran Derivative: Benzofuran derivatives are synthesized through cyclization reactions involving phenol and acetylene derivatives.
Coupling with Isophthalate Ester: The benzofuran derivative is then coupled with an isophthalate ester in the presence of a coupling agent such as dicyclohexylcarbodiimide (DCC) and a catalyst like 4-dimethylaminopyridine (DMAP).
Acetoxymethyl Esterification: The final step involves the esterification of the coupled product with acetoxymethyl chloride in the presence of a base such as triethylamine.
Industrial Production Methods
Industrial production of sodium-binding benzofuran isophthalate acetoxymethyl ester follows similar synthetic routes but on a larger scale. The process involves optimizing reaction conditions to maximize yield and purity. Key considerations include:
Reaction Temperature and Time: Controlling the temperature and reaction time to ensure complete conversion of reactants.
Purification: Using techniques such as column chromatography and recrystallization to purify the final product.
Quality Control: Implementing rigorous quality control measures to ensure the consistency and reliability of the product.
Chemical Reactions Analysis
Types of Reactions
Sodium-binding benzofuran isophthalate acetoxymethyl ester undergoes several types of chemical reactions, including:
Hydrolysis: The acetoxymethyl ester group can be hydrolyzed to form the corresponding carboxylic acid.
Oxidation: The benzofuran moiety can undergo oxidation reactions to form various oxidized derivatives.
Substitution: The compound can participate in substitution reactions, particularly at the benzofuran ring.
Common Reagents and Conditions
Hydrolysis: Typically carried out in the presence of a strong acid or base, such as hydrochloric acid or sodium hydroxide, under aqueous conditions.
Oxidation: Common oxidizing agents include potassium permanganate and chromium trioxide.
Substitution: Reagents such as halogens (chlorine, bromine) and nucleophiles (amines, thiols) are used in substitution reactions.
Major Products Formed
Hydrolysis: Forms the corresponding carboxylic acid.
Oxidation: Produces oxidized benzofuran derivatives.
Substitution: Yields substituted benzofuran products with various functional groups.
Scientific Research Applications
Sodium-binding benzofuran isophthalate acetoxymethyl ester has a wide range of scientific research applications, including:
Chemistry: Used as a fluorescent indicator for measuring sodium ion concentrations in chemical reactions and processes.
Biology: Employed in cellular studies to monitor intracellular sodium levels, particularly in neurons and muscle cells.
Medicine: Utilized in medical research to study sodium ion dynamics in various physiological and pathological conditions, such as hypertension and cardiac arrhythmias.
Industry: Applied in industrial processes that require precise measurement of sodium ion concentrations, such as water treatment and quality control in manufacturing
Comparison with Similar Compounds
Sodium-binding benzofuran isophthalate acetoxymethyl ester is unique in its high selectivity for sodium ions over potassium ions. Similar compounds include:
Potassium-binding benzofuran isophthalate (PBFI): Selective for potassium ions and used for measuring potassium ion concentrations.
Sodium Green: Another sodium indicator with different spectral properties.
CoroNa Green: A sodium indicator with higher sensitivity but lower selectivity compared to sodium-binding benzofuran isophthalate acetoxymethyl ester.
The uniqueness of sodium-binding benzofuran isophthalate acetoxymethyl ester lies in its combination of high selectivity for sodium ions and its ratiometric fluorescence properties, making it a valuable tool for precise and accurate measurement of sodium ion concentrations in various research applications .
Biological Activity
Sbfi-AM (Sodium Binding Benzofuran Isophthalate Acetoxymethyl Ester) is a cell-permeable fluorescent indicator specifically designed to measure intracellular sodium ion concentrations. This compound has garnered attention in various biological studies due to its selectivity for sodium ions over potassium ions, making it a valuable tool in cellular physiology and biochemistry.
- Chemical Name : Sodium Binding Benzofuran Isophthalate Acetoxymethyl Ester
- CAS Number : 129423-53-6
- Kd Values :
- Sodium (Na): 20 mM
- Potassium (K): 120 mM
These properties indicate a higher affinity for sodium ions, which is crucial for accurate measurements in biological systems where sodium plays a significant role in cellular processes .
This compound enters cells and is hydrolyzed by intracellular esterases to release the active form, SBFI. This active form binds selectively to sodium ions, allowing for the quantification of intracellular sodium concentrations through fluorescence measurements. The fluorescence intensity correlates with the concentration of sodium ions, enabling real-time monitoring of sodium dynamics within living cells.
Case Studies
-
Speract Response in Sea Urchin Sperm
A study investigated the use of this compound to measure changes in intracellular sodium levels during the speract response in sea urchin sperm. The experiment established optimal loading conditions and demonstrated that a loading time of 3 hours at 14 °C was sufficient to achieve reliable fluorescence readings. It was found that the resting intracellular sodium concentration was approximately 20 ± 8 mM, with significant increases observed upon stimulation with speract . -
Calcium and pH Correlation
The same study also correlated changes in intracellular sodium levels with calcium and pH variations induced by speract. This comprehensive approach highlighted the interconnectedness of these ions in regulating sperm motility and fertilization processes .
Fluorescence Measurement Protocol
The protocol for measuring intracellular sodium concentrations using this compound involves several key steps:
- Cell Preparation : Cells are incubated with this compound under specified conditions.
- Fluorescence Measurement : After loading, fluorescence is measured using a spectrophotometer at specific wavelengths (e.g., excitation at 340 nm and emission at 500 nm).
- Data Analysis : The fluorescence intensity is compared against standard curves to quantify intracellular sodium concentrations.
Comparative Studies
A comparative analysis of various cell types using this compound has shown consistent results across different biological systems, confirming its reliability as a sodium indicator. This includes studies on cardiac myocytes and neuronal cells, where fluctuations in intracellular sodium were linked to physiological responses such as contraction and action potential generation .
Data Table
Study Focus | Cell Type | Key Findings |
---|---|---|
Speract Response | Sea Urchin Sperm | Basal [Na] = 20 ± 8 mM; increased with speract stimulation |
Calcium Dynamics | Cardiac Myocytes | Correlation between [Na] and [Ca] during contraction |
Neuronal Activity | Neuronal Cells | Role of [Na] in action potential generation |
Properties
IUPAC Name |
bis(acetyloxymethyl) 4-[6-[13-[2-[2,4-bis(acetyloxymethoxycarbonyl)phenyl]-5-methoxy-1-benzofuran-6-yl]-1,4,10-trioxa-7,13-diazacyclopentadec-7-yl]-5-methoxy-1-benzofuran-2-yl]benzene-1,3-dicarboxylate | |
---|---|---|
Source | PubChem | |
URL | https://pubchem.ncbi.nlm.nih.gov | |
Description | Data deposited in or computed by PubChem | |
InChI |
InChI=1S/C56H58N2O23/c1-33(59)72-29-76-53(63)37-7-9-41(43(21-37)55(65)78-31-74-35(3)61)49-23-39-25-51(67-5)45(27-47(39)80-49)57-11-15-69-16-12-58(14-18-71-20-19-70-17-13-57)46-28-48-40(26-52(46)68-6)24-50(81-48)42-10-8-38(54(64)77-30-73-34(2)60)22-44(42)56(66)79-32-75-36(4)62/h7-10,21-28H,11-20,29-32H2,1-6H3 | |
Source | PubChem | |
URL | https://pubchem.ncbi.nlm.nih.gov | |
Description | Data deposited in or computed by PubChem | |
InChI Key |
MWUYLQGOGOLRQS-UHFFFAOYSA-N | |
Source | PubChem | |
URL | https://pubchem.ncbi.nlm.nih.gov | |
Description | Data deposited in or computed by PubChem | |
Canonical SMILES |
CC(=O)OCOC(=O)C1=CC(=C(C=C1)C2=CC3=CC(=C(C=C3O2)N4CCOCCN(CCOCCOCC4)C5=C(C=C6C=C(OC6=C5)C7=C(C=C(C=C7)C(=O)OCOC(=O)C)C(=O)OCOC(=O)C)OC)OC)C(=O)OCOC(=O)C | |
Source | PubChem | |
URL | https://pubchem.ncbi.nlm.nih.gov | |
Description | Data deposited in or computed by PubChem | |
Molecular Formula |
C56H58N2O23 | |
Source | PubChem | |
URL | https://pubchem.ncbi.nlm.nih.gov | |
Description | Data deposited in or computed by PubChem | |
DSSTOX Substance ID |
DTXSID70402219 | |
Record name | SBFI-AM | |
Source | EPA DSSTox | |
URL | https://comptox.epa.gov/dashboard/DTXSID70402219 | |
Description | DSSTox provides a high quality public chemistry resource for supporting improved predictive toxicology. | |
Molecular Weight |
1127.1 g/mol | |
Source | PubChem | |
URL | https://pubchem.ncbi.nlm.nih.gov | |
Description | Data deposited in or computed by PubChem | |
CAS No. |
129423-53-6 | |
Record name | 1,3-Benzenedicarboxylic acid, 4,4′-[1,4,10-trioxa-7,13-diazacyclopentadecane-7,13-diylbis(5-methoxy-6,2-benzofurandiyl)]bis-, 1,1′,3,3′-tetrakis[(acetyloxy)methyl] ester | |
Source | CAS Common Chemistry | |
URL | https://commonchemistry.cas.org/detail?cas_rn=129423-53-6 | |
Description | CAS Common Chemistry is an open community resource for accessing chemical information. Nearly 500,000 chemical substances from CAS REGISTRY cover areas of community interest, including common and frequently regulated chemicals, and those relevant to high school and undergraduate chemistry classes. This chemical information, curated by our expert scientists, is provided in alignment with our mission as a division of the American Chemical Society. | |
Explanation | The data from CAS Common Chemistry is provided under a CC-BY-NC 4.0 license, unless otherwise stated. | |
Record name | SBFI-AM | |
Source | EPA DSSTox | |
URL | https://comptox.epa.gov/dashboard/DTXSID70402219 | |
Description | DSSTox provides a high quality public chemistry resource for supporting improved predictive toxicology. | |
Retrosynthesis Analysis
AI-Powered Synthesis Planning: Our tool employs the Template_relevance Pistachio, Template_relevance Bkms_metabolic, Template_relevance Pistachio_ringbreaker, Template_relevance Reaxys, Template_relevance Reaxys_biocatalysis model, leveraging a vast database of chemical reactions to predict feasible synthetic routes.
One-Step Synthesis Focus: Specifically designed for one-step synthesis, it provides concise and direct routes for your target compounds, streamlining the synthesis process.
Accurate Predictions: Utilizing the extensive PISTACHIO, BKMS_METABOLIC, PISTACHIO_RINGBREAKER, REAXYS, REAXYS_BIOCATALYSIS database, our tool offers high-accuracy predictions, reflecting the latest in chemical research and data.
Strategy Settings
Precursor scoring | Relevance Heuristic |
---|---|
Min. plausibility | 0.01 |
Model | Template_relevance |
Template Set | Pistachio/Bkms_metabolic/Pistachio_ringbreaker/Reaxys/Reaxys_biocatalysis |
Top-N result to add to graph | 6 |
Feasible Synthetic Routes
Disclaimer and Information on In-Vitro Research Products
Please be aware that all articles and product information presented on BenchChem are intended solely for informational purposes. The products available for purchase on BenchChem are specifically designed for in-vitro studies, which are conducted outside of living organisms. In-vitro studies, derived from the Latin term "in glass," involve experiments performed in controlled laboratory settings using cells or tissues. It is important to note that these products are not categorized as medicines or drugs, and they have not received approval from the FDA for the prevention, treatment, or cure of any medical condition, ailment, or disease. We must emphasize that any form of bodily introduction of these products into humans or animals is strictly prohibited by law. It is essential to adhere to these guidelines to ensure compliance with legal and ethical standards in research and experimentation.