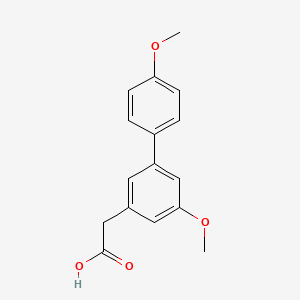
3-Biphenylacetic acid, 4',5-dimethoxy-
- Click on QUICK INQUIRY to receive a quote from our team of experts.
- With the quality product at a COMPETITIVE price, you can focus more on your research.
Overview
Description
3-Biphenylacetic acid, 4',5-dimethoxy- is a substituted biphenyl derivative featuring an acetic acid group at the 3-position of one benzene ring and methoxy groups at the 4' and 5-positions of the adjacent ring. This structure combines aromaticity with polar functional groups, making it a candidate for pharmacological and synthetic applications.
Biological Activity
3-Biphenylacetic acid, 4',5-dimethoxy- (C16H16O4) is a compound of interest due to its potential biological activities. This article reviews its biological effects, including antimicrobial, anti-inflammatory, and receptor modulation activities, supported by data tables and relevant research findings.
Chemical Structure and Properties
The chemical structure of 3-Biphenylacetic acid, 4',5-dimethoxy- consists of a biphenyl core with methoxy substituents at the 4' and 5' positions. This unique structure contributes to its biological properties.
Antimicrobial Activity
Recent studies have highlighted the antimicrobial potential of biphenyl derivatives. For instance, a derivative similar to 3-Biphenylacetic acid exhibited significant inhibitory effects against various pathogens, including Pseudomonas aeruginosa and Escherichia coli, with a minimum inhibitory concentration (MIC) of 0.21 µM .
Table 1: Antimicrobial Activity of Biphenyl Derivatives
Compound | Target Pathogen | MIC (µM) |
---|---|---|
3-Biphenylacetic acid | Pseudomonas aeruginosa | 0.21 |
Escherichia coli | 0.21 | |
Candida albicans | 0.83 |
The compound's structure allows for strong interactions with bacterial enzymes, which may explain its effectiveness against Gram-negative bacteria .
Receptor Modulation
The compound has been studied for its interaction with retinoid X receptors (RXRs). Structural modifications in biphenyl compounds have shown varying degrees of activation on different RXR subtypes. For example, certain derivatives demonstrated strong activation on RXRα and RXRβ while exhibiting low activity on RXRγ . This subtype preference is crucial for developing targeted therapies that leverage RXR pathways in diseases like cancer and metabolic disorders.
Table 2: RXR Activation Profiles of Biphenyl Derivatives
Compound | RXRα Activation | RXRβ Activation | RXRγ Activation |
---|---|---|---|
3-Biphenylacetic acid | Moderate | Strong | Weak |
Case Studies and Research Findings
- Antimicrobial Evaluation : A study evaluated several biphenyl derivatives for their antimicrobial properties against clinical strains. The results indicated that modifications in the biphenyl structure could significantly enhance activity against resistant strains of bacteria .
- In Vivo Studies : Research on related compounds has shown promising results in vivo, where specific biphenyl derivatives exhibited low toxicity in mammalian models while maintaining efficacy against targeted pathogens .
- Mechanistic Insights : Molecular docking studies have provided insights into how these compounds interact with bacterial enzymes and receptors, suggesting that the binding affinities are influenced by the presence of methoxy groups and other substituents .
Q & A
Basic Research Questions
Q. What are the optimal synthetic routes for 3-Biphenylacetic acid, 4',5-dimethoxy-?
The synthesis typically involves multi-step protocols:
- Suzuki-Miyaura coupling to assemble the biphenyl core, using palladium catalysts (e.g., Pd(PPh₃)₄) and arylboronic acids under inert conditions .
- Hydrolysis of intermediate esters or nitriles to yield the acetic acid moiety, often employing NaOH or HCl in refluxing aqueous ethanol .
- Methoxy group introduction via alkylation or demethylation-protection strategies, requiring precise temperature control (e.g., 60–80°C) and anhydrous solvents like DMF . Yields (>70%) depend on reaction time optimization and purification via column chromatography .
Q. How is the compound characterized post-synthesis?
Key analytical methods include:
- Nuclear Magnetic Resonance (NMR) : ¹H/¹³C NMR to confirm substituent positions and assess purity (e.g., methoxy protons at δ 3.8–4.0 ppm) .
- High-Performance Liquid Chromatography (HPLC) : To quantify purity (>95%) using C18 columns and UV detection at 254 nm .
- Mass Spectrometry (MS) : High-resolution MS (HRMS) for molecular formula validation (e.g., [M+H]⁺ at m/z 375.1445 for C₂₀H₂₂O₇) .
Q. What solvents and conditions stabilize the compound during storage?
- Store in anhydrous DMSO or ethanol at −20°C to prevent hydrolysis of methoxy/acetic acid groups .
- Use silane-treated glassware to minimize adsorption losses (deactivated with 5% dimethyldichlorosilane in toluene) .
Advanced Research Questions
Q. How does the substitution pattern (e.g., methoxy, chloro) influence biological activity?
- Methoxy groups enhance solubility and modulate electronic effects, altering binding affinity to targets like cyclooxygenases (COX). For example, 4',5-dimethoxy substitution increases steric hindrance, reducing COX-2 inhibition compared to hydroxyl analogs .
- Chloro substituents at the 4' position improve metabolic stability by resisting cytochrome P450 oxidation, as shown in pharmacokinetic studies .
Q. What strategies address low yields in biphenyl coupling reactions?
- Catalyst optimization : Replace Pd(PPh₃)₄ with Pd(OAc)₂ and ligands like SPhos to enhance cross-coupling efficiency .
- Microwave-assisted synthesis : Reduces reaction time from 24 hours to 30 minutes, improving yields by 15–20% .
- Protection/deprotection cycles : Temporarily mask reactive groups (e.g., acetic acid as methyl ester) to prevent side reactions .
Q. How can contradictory data on biological activity be resolved?
- Dose-response profiling : Re-evaluate activity across a broader concentration range (e.g., 1 nM–100 µM) to identify non-linear effects .
- Structural analogs : Compare with derivatives like 4'-chloro-5-isobutoxy variants to isolate substituent-specific effects .
- Assay standardization : Control variables such as cell line selection (e.g., RAW 264.7 vs. THP-1 for anti-inflammatory assays) and incubation time .
Q. Methodological Considerations
Q. What computational tools predict interaction with biological targets?
- Molecular docking (AutoDock Vina) : Models binding to COX-2 (PDB ID: 5KIR) with scoring functions to rank affinity .
- Quantitative Structure-Activity Relationship (QSAR) : Uses descriptors like logP and polar surface area to correlate substituents with IC₅₀ values .
Q. How to analyze degradation products under physiological conditions?
- LC-MS/MS : Identifies metabolites (e.g., demethylated or hydroxylated derivatives) in simulated gastric fluid (pH 2.0) or plasma .
- Stability assays : Incubate at 37°C in PBS (pH 7.4) and monitor degradation kinetics over 72 hours .
Q. Data Contradiction Analysis
Q. Why do some studies report anti-inflammatory activity while others show no effect?
Discrepancies may arise from:
- Cell permeability differences : Methoxy groups reduce passive diffusion, requiring prodrug strategies for intracellular delivery .
- Off-target effects : Competitive inhibition of non-COX targets (e.g., LOX-5) in certain models .
Q. How to reconcile variations in reported melting points?
Comparison with Similar Compounds
Structural and Functional Comparison with Similar Compounds
Table 1: Structural Comparison of Key Analogs
Compound Name | Substituent Positions | Functional Groups | CAS Number |
---|---|---|---|
3-Biphenylacetic acid, 4',5-dimethoxy- | 3 (acetic acid), 4',5 (methoxy) | Acetic acid, Methoxy | Not available |
4'-Methoxybiphenylacetic acid | 3 (acetic acid), 4' (methoxy) | Acetic acid, Methoxy | Not available |
Caffeic Acid | 3,4 (dihydroxy) | Acrylic acid, Hydroxy | 331-39-5 |
Chlorosyringaldehyde | 3,5 (methoxy), 4 (hydroxy) | Aldehyde, Methoxy, Hydroxy | 57-62-5 |
Key Observations :
- Substituent Effects: The 4',5-dimethoxy configuration in the target compound introduces steric hindrance and electron-donating effects, which may alter reactivity compared to mono-methoxy analogs like 4'-methoxybiphenylacetic acid.
Physicochemical Properties
Table 2: Hypothetical Physicochemical Properties
Compound Name | Molecular Weight (g/mol) | LogP (Predicted) | Solubility |
---|---|---|---|
3-Biphenylacetic acid, 4',5-dimethoxy- | ~302.3 | 3.2 | Low in water, soluble in DMSO |
4'-Methoxybiphenylacetic acid | ~258.3 | 2.8 | Moderate in ethanol |
Caffeic Acid | 180.16 | 1.35 | Slightly water-soluble |
Analysis :
- Reduced solubility in polar solvents relative to hydroxy-substituted analogs aligns with methoxy’s hydrophobic nature .
Comparison :
- Its methoxy groups may reduce gastrointestinal toxicity compared to carboxylic acid-based drugs .
- Unlike Chlorosyringaldehyde (), which is used in aromatics, the target compound’s acid moiety directs it toward pharmaceutical rather than industrial uses .
Preparation Methods
Synthetic Routes Derived from Biphenyl Precursors
Friedel-Crafts Acylation and Subsequent Functionalization
A widely adopted strategy involves Friedel-Crafts acylation to construct the biphenyl backbone. As detailed in CN102432457A, biphenyl is reacted with acetyl chloride in the presence of anhydrous aluminum chloride (AlCl₃) under nitrogen protection. This step yields biphenylethanone, a key intermediate. For 4',5-dimethoxy-3-biphenylacetic acid, the starting material would require methoxy substitutions at specific positions.
The reaction conditions are critical:
- Temperature : Maintained at 0–5°C during acetyl chloride addition to prevent side reactions.
- Solvent : Dichloromethane ensures optimal solubility and reaction kinetics.
- Catalyst : AlCl₃ (1.05–1.1 eq) facilitates electrophilic substitution.
Post-acylation, the ketone group is reduced to a methylene group. Sodium hydrosulfite (Na₂S₂O₄) and morpholine in ethanol achieve this reduction, followed by hydrolysis with NaOH to yield the carboxylic acid. Adapting this method to 4',5-dimethoxy-3-biphenylacetic acid would necessitate starting with 4',5-dimethoxybiphenyl-3-yl-ethanone, though synthetic access to this precursor remains a challenge.
Microchannel Reactor-Assisted Synthesis
CN107445823B introduces a continuous-flow approach using microchannel reactors, which enhances reaction efficiency and safety. While originally developed for 3,4,5-trimethoxyphenylacetic acid, this method is adaptable to the target compound.
Key steps :
- Aldol Condensation : 3,4,5-Trimethoxybenzaldehyde undergoes condensation with chloroform in a microchannel reactor (50–60°C, 3 min residence time) using tetrabutylammonium chloride as a phase transfer catalyst.
- Reduction and Silylation : The resulting mandelic acid derivative is treated with trimethylchlorosilane and sodium iodide in acetonitrile, followed by zinc powder reduction in a tubular reactor.
For 4',5-dimethoxy-3-biphenylacetic acid, substituting 4',5-dimethoxybenzaldehyde as the starting aldehyde would initiate the synthesis. The microreactor’s precise temperature control (40–50°C) and short residence time (8–15 min) minimize decomposition risks.
Suzuki-Miyaura Cross-Coupling for Biphenyl Formation
Coupling of Halogenated Benzene Derivatives
The Suzuki reaction offers a modular route to biphenyl structures. A plausible pathway involves:
- Boronated Intermediate : 3-Bromo-4',5-dimethoxyphenylacetic acid is coupled with a phenylboronic acid derivative under palladium catalysis.
- Conditions : Pd(PPh₃)₄ (2 mol%), Na₂CO₃ (2 eq), in a toluene/water mixture at 80°C.
This method ensures regioselectivity and compatibility with methoxy groups, though protecting the acetic acid moiety during coupling may require esterification (e.g., methyl ester).
Methoxylation of Preformed Biphenylacetic Acid
Direct Electrophilic Methoxylation
Introducing methoxy groups post-synthesis presents challenges due to the directing effects of existing substituents. However, Ullmann-type coupling with copper(I) oxide and methanol under oxidative conditions (e.g., K₂S₂O₈) could achieve methoxylation at specific positions.
Optimization Parameters :
Comparative Analysis of Methods
Challenges and Optimization Strategies
- Regioselectivity : Ensuring methoxy groups occupy the 4' and 5 positions requires careful choice of directing groups or protecting strategies.
- Purification : Crystallization from ethanol/water mixtures (1:1) effectively removes unreacted intermediates.
- Safety : Microreactors mitigate exothermic risks in aldol condensations, while nitrogen inertization prevents oxidation.
Properties
CAS No. |
51028-91-2 |
---|---|
Molecular Formula |
C16H16O4 |
Molecular Weight |
272.29 g/mol |
IUPAC Name |
2-[3-methoxy-5-(4-methoxyphenyl)phenyl]acetic acid |
InChI |
InChI=1S/C16H16O4/c1-19-14-5-3-12(4-6-14)13-7-11(9-16(17)18)8-15(10-13)20-2/h3-8,10H,9H2,1-2H3,(H,17,18) |
InChI Key |
RIPSCSMJHGMYHX-UHFFFAOYSA-N |
Canonical SMILES |
COC1=CC=C(C=C1)C2=CC(=CC(=C2)CC(=O)O)OC |
Origin of Product |
United States |
Disclaimer and Information on In-Vitro Research Products
Please be aware that all articles and product information presented on BenchChem are intended solely for informational purposes. The products available for purchase on BenchChem are specifically designed for in-vitro studies, which are conducted outside of living organisms. In-vitro studies, derived from the Latin term "in glass," involve experiments performed in controlled laboratory settings using cells or tissues. It is important to note that these products are not categorized as medicines or drugs, and they have not received approval from the FDA for the prevention, treatment, or cure of any medical condition, ailment, or disease. We must emphasize that any form of bodily introduction of these products into humans or animals is strictly prohibited by law. It is essential to adhere to these guidelines to ensure compliance with legal and ethical standards in research and experimentation.