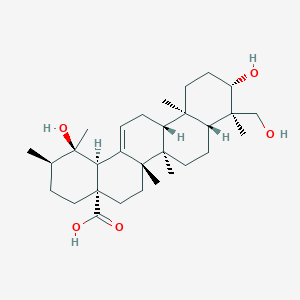
Rotundic acid
Overview
Description
Rotundic acid is a triterpenoid found in the leaves of Ilex pubescens Hook . It exhibits potent tumor cell growth inhibiting properties and has shown cytotoxic activities towards various cancer cell lines . It is also found in Euscaphis japonica .
Synthesis Analysis
Rotundic acid has been found to improve nonalcoholic steatohepatitis in mice by regulating glycolysis and the TLR4/AP1 signaling pathway . There have been studies on the synthesis of rotundic acid derivatives, which were modified at the 28-COOH position .Molecular Structure Analysis
The molecular formula of rotundic acid is C30H48O5 . The structure of rotundic acid was confirmed by IR, MS, 1H NMR and 13C NMR .Chemical Reactions Analysis
Rotundic acid has been found to regulate triglyceride metabolism and glycerophospholipid metabolism, restore gut microbiota structure, and increase the relative abundance of SCFAs‐producing bacteria to exert its hypolipidemic effects .Physical And Chemical Properties Analysis
Rotundic acid has a molecular weight of 488.70 .Scientific Research Applications
Rotundic Acid in Cancer Research
Rotundic acid (RA) and its synthetic derivatives have shown promising anti-cancer properties in preclinical pharmacological test systems against breast cancer, colorectal cancer, liver cancer, and cervical cancer. The mechanisms include inducing apoptosis, inhibiting oxidative stress, anti-inflammatory actions, cytotoxicity, cell cycle arrest, anti-proliferative effects, anti-angiogenesis, and inhibiting the migration and invasion of cancer cells .
Rotundic Acid as an Anti-Obesity Agent
RA has been identified as an anti-obesity agent in mice induced with obesity by a high-fat diet. It acts by modulating metabolic pathways associated with obesity .
Rotundic Acid in Cardiovascular Disease Prevention
New derivatives of rotundic acid have been synthesized and characterized for their potential application in the prevention of cardiovascular diseases .
Rotundic Acid in Metabolic Syndrome Protection
RA is a major triterpene constituent in Ilex rotunda Thunb., whose barks are used to make herbal tea for health care in southern China. It has been studied for its protective effects against metabolic syndrome .
Rotundic Acid in Nonalcoholic Steatohepatitis (NASH) Treatment RA is key in lowering lipid content and alleviating inflammation, which are crucial in the development and progression of NASH. The study aims to systematically evaluate the potential mechanisms through which RA affects NASH .
Mechanism of Action
Rotundic acid (RA) is a triterpenoid compound found in Ilicis Rotundae Cortex, known for its diverse pharmacological properties . This article aims to provide a comprehensive overview of the mechanism of action of RA, focusing on its targets, mode of action, biochemical pathways, pharmacokinetics, results of action, and the influence of environmental factors.
Target of Action
The primary targets of RA are the Toll-like receptor 4 (TLR4)/activator protein-1 (AP1) signaling pathway and the AKT/mTOR and MAPK pathways . These pathways play crucial roles in inflammation, cell proliferation, and apoptosis .
Mode of Action
RA interacts with its targets to induce significant changes in cellular processes. It attenuates the TLR4/AP1 pathway and reverses glycolysis, thereby reducing lipid accumulation and inflammation . Additionally, RA induces DNA damage, cell cycle arrest, and apoptosis by modulating the AKT/mTOR and MAPK pathways .
Biochemical Pathways
RA affects two main biochemical pathways: the TLR4/AP1 signaling pathway and glycolysis . By reversing glycolysis and attenuating the TLR4/AP1 pathway, RA reduces lipid accumulation and inflammation, which are key factors in conditions like nonalcoholic steatohepatitis (NASH) .
Result of Action
The molecular and cellular effects of RA’s action are multifaceted. It has been shown to lower lipid content, alleviate inflammation , induce DNA damage, cause cell cycle arrest, and trigger apoptosis . These effects contribute to RA’s anticancer activity against hepatocellular carcinoma (HCC) and its potential therapeutic effects on NASH .
Action Environment
Environmental factors can influence the action, efficacy, and stability of RA. For instance, diet, a key environmental factor, can affect the development and progression of diseases like NASH, where RA has shown therapeutic potential . .
Safety and Hazards
Future Directions
Rotundic acid and its synthetic derivatives possess promising anti-cancer properties against breast, colorectal, liver, and cervical cancers in various preclinical pharmacological test systems . It has been proposed that rotundic acid and its derived compounds have the capability to serve as a hopeful chemotherapeutic agent, so further extensive clinical research is necessary .
properties
IUPAC Name |
(1R,2R,4aS,6aR,6aS,6bR,8aR,9R,10S,12aR,14bS)-1,10-dihydroxy-9-(hydroxymethyl)-1,2,6a,6b,9,12a-hexamethyl-2,3,4,5,6,6a,7,8,8a,10,11,12,13,14b-tetradecahydropicene-4a-carboxylic acid | |
---|---|---|
Source | PubChem | |
URL | https://pubchem.ncbi.nlm.nih.gov | |
Description | Data deposited in or computed by PubChem | |
InChI |
InChI=1S/C30H48O5/c1-18-9-14-30(24(33)34)16-15-27(4)19(23(30)29(18,6)35)7-8-21-25(2)12-11-22(32)26(3,17-31)20(25)10-13-28(21,27)5/h7,18,20-23,31-32,35H,8-17H2,1-6H3,(H,33,34)/t18-,20-,21-,22+,23-,25+,26+,27-,28-,29-,30+/m1/s1 | |
Source | PubChem | |
URL | https://pubchem.ncbi.nlm.nih.gov | |
Description | Data deposited in or computed by PubChem | |
InChI Key |
YLHQFGOOMKJFLP-LTFXOGOQSA-N | |
Source | PubChem | |
URL | https://pubchem.ncbi.nlm.nih.gov | |
Description | Data deposited in or computed by PubChem | |
Canonical SMILES |
CC1CCC2(CCC3(C(=CCC4C3(CCC5C4(CCC(C5(C)CO)O)C)C)C2C1(C)O)C)C(=O)O | |
Source | PubChem | |
URL | https://pubchem.ncbi.nlm.nih.gov | |
Description | Data deposited in or computed by PubChem | |
Isomeric SMILES |
C[C@@H]1CC[C@@]2(CC[C@@]3(C(=CC[C@H]4[C@]3(CC[C@@H]5[C@@]4(CC[C@@H]([C@@]5(C)CO)O)C)C)[C@@H]2[C@]1(C)O)C)C(=O)O | |
Source | PubChem | |
URL | https://pubchem.ncbi.nlm.nih.gov | |
Description | Data deposited in or computed by PubChem | |
Molecular Formula |
C30H48O5 | |
Source | PubChem | |
URL | https://pubchem.ncbi.nlm.nih.gov | |
Description | Data deposited in or computed by PubChem | |
DSSTOX Substance ID |
DTXSID80173963 | |
Record name | Urs-12-en-28-oic acid, 3,19,23-trihydroxy-, (3beta,4alpha)- | |
Source | EPA DSSTox | |
URL | https://comptox.epa.gov/dashboard/DTXSID80173963 | |
Description | DSSTox provides a high quality public chemistry resource for supporting improved predictive toxicology. | |
Molecular Weight |
488.7 g/mol | |
Source | PubChem | |
URL | https://pubchem.ncbi.nlm.nih.gov | |
Description | Data deposited in or computed by PubChem | |
Product Name |
Rotundic acid | |
CAS RN |
20137-37-5 | |
Record name | Rotundic acid | |
Source | CAS Common Chemistry | |
URL | https://commonchemistry.cas.org/detail?cas_rn=20137-37-5 | |
Description | CAS Common Chemistry is an open community resource for accessing chemical information. Nearly 500,000 chemical substances from CAS REGISTRY cover areas of community interest, including common and frequently regulated chemicals, and those relevant to high school and undergraduate chemistry classes. This chemical information, curated by our expert scientists, is provided in alignment with our mission as a division of the American Chemical Society. | |
Explanation | The data from CAS Common Chemistry is provided under a CC-BY-NC 4.0 license, unless otherwise stated. | |
Record name | Urs-12-en-28-oic acid, 3,19,23-trihydroxy-, (3beta,4alpha)- | |
Source | ChemIDplus | |
URL | https://pubchem.ncbi.nlm.nih.gov/substance/?source=chemidplus&sourceid=0020137375 | |
Description | ChemIDplus is a free, web search system that provides access to the structure and nomenclature authority files used for the identification of chemical substances cited in National Library of Medicine (NLM) databases, including the TOXNET system. | |
Record name | Urs-12-en-28-oic acid, 3,19,23-trihydroxy-, (3beta,4alpha)- | |
Source | EPA DSSTox | |
URL | https://comptox.epa.gov/dashboard/DTXSID80173963 | |
Description | DSSTox provides a high quality public chemistry resource for supporting improved predictive toxicology. | |
Retrosynthesis Analysis
AI-Powered Synthesis Planning: Our tool employs the Template_relevance Pistachio, Template_relevance Bkms_metabolic, Template_relevance Pistachio_ringbreaker, Template_relevance Reaxys, Template_relevance Reaxys_biocatalysis model, leveraging a vast database of chemical reactions to predict feasible synthetic routes.
One-Step Synthesis Focus: Specifically designed for one-step synthesis, it provides concise and direct routes for your target compounds, streamlining the synthesis process.
Accurate Predictions: Utilizing the extensive PISTACHIO, BKMS_METABOLIC, PISTACHIO_RINGBREAKER, REAXYS, REAXYS_BIOCATALYSIS database, our tool offers high-accuracy predictions, reflecting the latest in chemical research and data.
Strategy Settings
Precursor scoring | Relevance Heuristic |
---|---|
Min. plausibility | 0.01 |
Model | Template_relevance |
Template Set | Pistachio/Bkms_metabolic/Pistachio_ringbreaker/Reaxys/Reaxys_biocatalysis |
Top-N result to add to graph | 6 |
Feasible Synthetic Routes
Q & A
Q1: What are the primary molecular targets of Rotundic acid?
A1: Rotundic acid has been shown to interact with various molecular targets, influencing different cellular processes. Notably, it acts as a noncompetitive inhibitor of protein tyrosine phosphatase 1B (PTP1B) and T-cell PTP (TCPTP) by interacting with their C-terminus []. This inhibition enhances leptin sensitivity, contributing to its anti-obesity and anti-aging effects. Additionally, RA has been shown to modulate the ATM/p53 pathway, leading to enhanced apoptosis in cancer cells when combined with radiation therapy []. It also interacts with TLR4, blocking its dimerization and subsequently suppressing inflammatory responses [].
Q2: What is the role of Rotundic acid in regulating the Bcl-2 family proteins?
A2: Research suggests that Rotundic acid can influence the balance of pro-apoptotic and anti-apoptotic proteins within the Bcl-2 family. In alcoholic hepatitis models, RA treatment increased Bcl-2 levels while decreasing Bax and caspase-3 levels []. This shift in the Bcl-2/Bax ratio indicates a protective effect against apoptosis. Conversely, in MCF-7 breast cancer cells, RA combined with radiation therapy induced Bcl-2-associated X protein expression, promoting apoptosis []. These findings suggest that RA's effects on Bcl-2 family proteins are context-dependent and may vary across cell types and disease models.
Q3: How does Rotundic acid contribute to angiogenesis?
A3: Rotundic acid exhibits pro-angiogenic properties by promoting cell proliferation, adhesion, migration, and tube formation in human umbilical vein endothelial cells (HUVECs) []. This effect is mediated, at least in part, through the activation of the JAK-STAT3 signaling pathway.
Q4: Can Rotundic acid influence gut microbiota composition?
A4: Emerging evidence suggests that Rotundic acid can modulate the gut microbiome. In type 2 diabetes rat models, RA treatment increased the abundance of beneficial bacteria like Prevotella, Ruminococcus, Leuconostoc, and Streptococcus, while decreasing opportunistic pathogens like Klebsiella and Proteus []. These alterations in gut microbiota composition were correlated with improvements in various metabolic parameters, suggesting a potential role for RA in ameliorating metabolic dysfunction through its influence on the gut microbiome.
Q5: What is the molecular formula and weight of Rotundic acid?
A5: Rotundic acid has the molecular formula C30H48O3 and a molecular weight of 456.7 g/mol.
Q6: How do structural modifications of Rotundic acid affect its biological activity?
A6: Modifying Rotundic acid at the 28-COOH position has been explored to enhance its properties. For instance, introducing amino acid moieties at this position led to derivatives with improved cytotoxicity against various cancer cell lines []. Specifically, compound 5a, an amino acid derivative of RA, displayed greater potency compared to the parent compound. This suggests that structural modifications can significantly impact the biological activity of RA and highlights the potential for developing more potent derivatives for therapeutic applications.
Q7: What strategies have been explored to improve the stability, solubility, or bioavailability of Rotundic acid?
A7: One approach to enhance the pharmacokinetic properties of Rotundic acid involves formulating it into inclusion complexes. Specifically, a beta-cyclodextrin polymer inclusion complex of pedunculoside, a Rotundic acid derivative, was developed to address its limitations of poor bioavailability and rapid elimination []. This complex effectively increased plasma exposure and reduced intestinal metabolism, highlighting the potential of such formulation strategies for improving the therapeutic efficacy of RA.
Q8: What is known about the absorption, distribution, metabolism, and excretion (ADME) of Rotundic acid?
A8: Research indicates that Rotundic acid might be a substrate for P-glycoprotein (P-gp), an efflux transporter, which could contribute to its limited oral bioavailability []. Additionally, RA is primarily metabolized by CYP3A4, a major drug-metabolizing enzyme in the liver. Co-administration of verapamil, a P-gp inhibitor, significantly increased the plasma concentration and area under the curve (AUC) of RA in rats, while reducing its oral clearance. This suggests that inhibiting P-gp could be a strategy to enhance RA's bioavailability.
Disclaimer and Information on In-Vitro Research Products
Please be aware that all articles and product information presented on BenchChem are intended solely for informational purposes. The products available for purchase on BenchChem are specifically designed for in-vitro studies, which are conducted outside of living organisms. In-vitro studies, derived from the Latin term "in glass," involve experiments performed in controlled laboratory settings using cells or tissues. It is important to note that these products are not categorized as medicines or drugs, and they have not received approval from the FDA for the prevention, treatment, or cure of any medical condition, ailment, or disease. We must emphasize that any form of bodily introduction of these products into humans or animals is strictly prohibited by law. It is essential to adhere to these guidelines to ensure compliance with legal and ethical standards in research and experimentation.