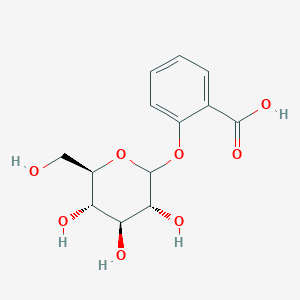
Salicylic acid beta-D-glucoside
Overview
Description
Salicylic acid beta-D-glucoside, also known as salicylic acid 2-O-beta-D-glucoside, is a naturally occurring compound found in plants. It is a derivative of salicylic acid, a well-known plant hormone involved in various physiological processes, including plant defense mechanisms against pathogens and stress responses. This compound is formed by the conjugation of salicylic acid with glucose, resulting in a more stable and water-soluble form.
Mechanism of Action
Target of Action
Salicylic Acid Beta-D-Glucoside (SAG) is the predominant glycosylated metabolite of Salicylic Acid (SA) . SA plays a crucial role in plant resilience under unfavorable environments . It is involved in the induction of systemic acquired resistance (SAR) against pathogens .
Mode of Action
SAG is involved in the modulation of the salicylic acid (SA) signaling pathway . It is known to induce a slow and long-lasting generation of reactive oxygen species . The expression of genes involved in SA pathway activation and subsequent modification, as well as, associated SA metabolites are regulated by SAG .
Biochemical Pathways
SAG affects the SA biosynthesis pathways and regulates their products under several abiotic stresses . It is involved in redox signaling, cross-talks with other plant hormones, and mitogen-activated protein kinase pathways . It also plays a role in the regulation of the expression levels of genes in the SA biosynthetic pathway .
Pharmacokinetics
It is known that sag is a metabolite of sa and its accumulation in plants can be influenced by various factors .
Result of Action
The action of SAG results in the modulation of the SA signaling pathway, leading to the induction of systemic acquired resistance (SAR) against pathogens . It also influences the expression of genes involved in SA pathway activation and subsequent modification .
Action Environment
The action of SAG is influenced by environmental factors such as temperature, drought, and salinity . These abiotic stresses impose several challenges on crop production systems, and SAG plays a crucial role in plant resilience under these unfavorable conditions .
Biochemical Analysis
Biochemical Properties
Salicylic Acid Beta-D-Glucoside interacts with various enzymes, proteins, and other biomolecules in biochemical reactions. It is synthesized through two distinct and compartmentalized pathways that employ different precursors: the phenylpropanoid route in the cytoplasm initiates from phenylalanine, and the isochorismate pathway takes place in the chloroplast . Most of the SA synthesized in plants is glucosylated to form SAG . These conjugation reactions are catalyzed by cytosolic SA glucosyltransferases .
Cellular Effects
This compound has significant effects on various types of cells and cellular processes. It influences cell function by impacting cell signaling pathways, gene expression, and cellular metabolism . For instance, it plays a crucial role in the regulation of physiological and biochemical processes during the entire lifespan of the plant .
Molecular Mechanism
The molecular mechanism of action of this compound involves its binding interactions with biomolecules, enzyme inhibition or activation, and changes in gene expression . It exerts its effects at the molecular level, including any binding interactions with biomolecules, enzyme inhibition or activation, and changes in gene expression .
Temporal Effects in Laboratory Settings
In laboratory settings, the effects of this compound change over time. It has been observed that the accumulation of SAG significantly increased in mature leaves of plants exposed for 150 days of continuous feeding compared to control plants .
Dosage Effects in Animal Models
While specific studies on the dosage effects of this compound in animal models are limited, it’s worth noting that its parent compound, Salicylic Acid, and its derivatives have been extensively studied and used in human medicine for their anti-inflammatory and analgesic effects .
Metabolic Pathways
This compound is involved in the metabolic pathways of Salicylic Acid. It is synthesized through the phenylpropanoid route or the isochorismate pathway . The glucose conjugation at the hydroxyl group of SA results in the formation of SAG .
Transport and Distribution
This compound is transported and distributed within cells and tissues. SAG is sequestered in the vacuole by an ATP-binding cassette transporter mechanism or an H+ -antiporter mechanism . Free SA is mobile and can be transported within the plant, mainly via the phloem .
Subcellular Localization
The subcellular localization of this compound is primarily in the cytoplasm . This localization plays a crucial role in its activity and function, contributing to the accumulation of defense metabolites like lignin and DIMBOA .
Preparation Methods
Synthetic Routes and Reaction Conditions: Salicylic acid beta-D-glucoside can be synthesized through enzymatic or chemical methods. The enzymatic method involves the use of glucosyltransferases, which catalyze the transfer of glucose from a donor molecule to salicylic acid. This process typically occurs under mild conditions, such as a pH of 7-8 and temperatures around 25-30°C .
Industrial Production Methods: Industrial production of this compound often employs microbial fermentation techniques. For instance, genetically engineered strains of Escherichia coli can be used to produce the compound by introducing genes encoding the necessary enzymes for salicylic acid and glucose conjugation . This method is environmentally friendly and can be scaled up for large-scale production.
Chemical Reactions Analysis
Types of Reactions: Salicylic acid beta-D-glucoside undergoes various chemical reactions, including hydrolysis, oxidation, and conjugation.
Common Reagents and Conditions:
Hydrolysis: This reaction involves breaking the glycosidic bond to release free salicylic acid and glucose. It can be catalyzed by enzymes such as beta-glucosidase or by acidic conditions.
Major Products:
Hydrolysis: Salicylic acid and glucose.
Oxidation: Dihydroxybenzoic acids (e.g., gentisic acid).
Scientific Research Applications
Salicylic acid beta-D-glucoside has a wide range of applications in scientific research:
Chemistry: It is used as a model compound to study glycosylation reactions and the stability of glycosidic bonds.
Comparison with Similar Compounds
Salicylic acid beta-D-glucoside is unique due to its glycosylated form, which enhances its stability and solubility compared to free salicylic acid. Similar compounds include:
Salicylic acid glucose ester: Another glycosylated form of salicylic acid, but with glucose attached to the carboxyl group instead of the hydroxyl group.
Calycosin-7-O-beta-D-glucoside: An isoflavonoid glucoside found in Astragalus membranaceus, which also exhibits beneficial health properties.
These compounds share the common feature of being glycosylated derivatives of phenolic acids or flavonoids, which generally enhance their stability and bioavailability.
Properties
IUPAC Name |
2-[3,4,5-trihydroxy-6-(hydroxymethyl)oxan-2-yl]oxybenzoic acid | |
---|---|---|
Details | Computed by LexiChem 2.6.6 (PubChem release 2019.06.18) | |
Source | PubChem | |
URL | https://pubchem.ncbi.nlm.nih.gov | |
Description | Data deposited in or computed by PubChem | |
InChI |
InChI=1S/C13H16O8/c14-5-8-9(15)10(16)11(17)13(21-8)20-7-4-2-1-3-6(7)12(18)19/h1-4,8-11,13-17H,5H2,(H,18,19) | |
Details | Computed by InChI 1.0.5 (PubChem release 2019.06.18) | |
Source | PubChem | |
URL | https://pubchem.ncbi.nlm.nih.gov | |
Description | Data deposited in or computed by PubChem | |
InChI Key |
TZPBMNKOLMSJPF-UHFFFAOYSA-N | |
Details | Computed by InChI 1.0.5 (PubChem release 2019.06.18) | |
Source | PubChem | |
URL | https://pubchem.ncbi.nlm.nih.gov | |
Description | Data deposited in or computed by PubChem | |
Canonical SMILES |
C1=CC=C(C(=C1)C(=O)O)OC2C(C(C(C(O2)CO)O)O)O | |
Details | Computed by OEChem 2.1.5 (PubChem release 2019.06.18) | |
Source | PubChem | |
URL | https://pubchem.ncbi.nlm.nih.gov | |
Description | Data deposited in or computed by PubChem | |
Molecular Formula |
C13H16O8 | |
Details | Computed by PubChem 2.1 (PubChem release 2019.06.18) | |
Source | PubChem | |
URL | https://pubchem.ncbi.nlm.nih.gov | |
Description | Data deposited in or computed by PubChem | |
DSSTOX Substance ID |
DTXSID50902207 | |
Record name | NoName_1429 | |
Source | EPA DSSTox | |
URL | https://comptox.epa.gov/dashboard/DTXSID50902207 | |
Description | DSSTox provides a high quality public chemistry resource for supporting improved predictive toxicology. | |
Molecular Weight |
300.26 g/mol | |
Details | Computed by PubChem 2.1 (PubChem release 2021.05.07) | |
Source | PubChem | |
URL | https://pubchem.ncbi.nlm.nih.gov | |
Description | Data deposited in or computed by PubChem | |
Physical Description |
Solid | |
Record name | Salicylic acid beta-D-glucoside | |
Source | Human Metabolome Database (HMDB) | |
URL | http://www.hmdb.ca/metabolites/HMDB0041271 | |
Description | The Human Metabolome Database (HMDB) is a freely available electronic database containing detailed information about small molecule metabolites found in the human body. | |
Explanation | HMDB is offered to the public as a freely available resource. Use and re-distribution of the data, in whole or in part, for commercial purposes requires explicit permission of the authors and explicit acknowledgment of the source material (HMDB) and the original publication (see the HMDB citing page). We ask that users who download significant portions of the database cite the HMDB paper in any resulting publications. | |
CAS No. |
10366-91-3 | |
Record name | Salicylic acid beta-D-glucoside | |
Source | Human Metabolome Database (HMDB) | |
URL | http://www.hmdb.ca/metabolites/HMDB0041271 | |
Description | The Human Metabolome Database (HMDB) is a freely available electronic database containing detailed information about small molecule metabolites found in the human body. | |
Explanation | HMDB is offered to the public as a freely available resource. Use and re-distribution of the data, in whole or in part, for commercial purposes requires explicit permission of the authors and explicit acknowledgment of the source material (HMDB) and the original publication (see the HMDB citing page). We ask that users who download significant portions of the database cite the HMDB paper in any resulting publications. | |
Melting Point |
162 - 165 °C | |
Record name | Salicylic acid beta-D-glucoside | |
Source | Human Metabolome Database (HMDB) | |
URL | http://www.hmdb.ca/metabolites/HMDB0041271 | |
Description | The Human Metabolome Database (HMDB) is a freely available electronic database containing detailed information about small molecule metabolites found in the human body. | |
Explanation | HMDB is offered to the public as a freely available resource. Use and re-distribution of the data, in whole or in part, for commercial purposes requires explicit permission of the authors and explicit acknowledgment of the source material (HMDB) and the original publication (see the HMDB citing page). We ask that users who download significant portions of the database cite the HMDB paper in any resulting publications. | |
Retrosynthesis Analysis
AI-Powered Synthesis Planning: Our tool employs the Template_relevance Pistachio, Template_relevance Bkms_metabolic, Template_relevance Pistachio_ringbreaker, Template_relevance Reaxys, Template_relevance Reaxys_biocatalysis model, leveraging a vast database of chemical reactions to predict feasible synthetic routes.
One-Step Synthesis Focus: Specifically designed for one-step synthesis, it provides concise and direct routes for your target compounds, streamlining the synthesis process.
Accurate Predictions: Utilizing the extensive PISTACHIO, BKMS_METABOLIC, PISTACHIO_RINGBREAKER, REAXYS, REAXYS_BIOCATALYSIS database, our tool offers high-accuracy predictions, reflecting the latest in chemical research and data.
Strategy Settings
Precursor scoring | Relevance Heuristic |
---|---|
Min. plausibility | 0.01 |
Model | Template_relevance |
Template Set | Pistachio/Bkms_metabolic/Pistachio_ringbreaker/Reaxys/Reaxys_biocatalysis |
Top-N result to add to graph | 6 |
Feasible Synthetic Routes
Q1: What does the research reveal about the role of salicylic acid beta-D-glucoside in rose plants infected with powdery mildew?
A1: The research demonstrates that this compound levels significantly increase in Rosa chinensis upon infection with the powdery mildew fungus, Podosphaera pannosa []. This increase mirrors the response observed when rose plants are treated with exogenous salicylic acid, a key hormone involved in plant defense signaling. These findings suggest that this compound plays a role in the plant's defense response against powdery mildew, potentially acting as a storage or signaling molecule related to salicylic acid.
Disclaimer and Information on In-Vitro Research Products
Please be aware that all articles and product information presented on BenchChem are intended solely for informational purposes. The products available for purchase on BenchChem are specifically designed for in-vitro studies, which are conducted outside of living organisms. In-vitro studies, derived from the Latin term "in glass," involve experiments performed in controlled laboratory settings using cells or tissues. It is important to note that these products are not categorized as medicines or drugs, and they have not received approval from the FDA for the prevention, treatment, or cure of any medical condition, ailment, or disease. We must emphasize that any form of bodily introduction of these products into humans or animals is strictly prohibited by law. It is essential to adhere to these guidelines to ensure compliance with legal and ethical standards in research and experimentation.