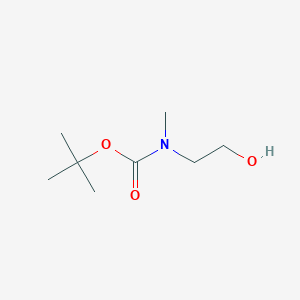
N-Boc-N-methyl-aminoethanol
Overview
Description
N-Boc-N-methyl-aminoethanol, also known as N-(tert-Butoxycarbonyl)-N-methylethanolamine, is an organic compound with the molecular formula C8H17NO3 and a molecular weight of 175.23 g/mol . It is commonly used as a protecting group in organic synthesis, particularly in the synthesis of peptides and other complex molecules. The compound appears as a colorless to pale yellow liquid and is slightly soluble in water but more soluble in organic solvents like chloroform and methanol .
Mechanism of Action
N-Boc-N-methyl-aminoethanol, also known as tert-Butyl (2-hydroxyethyl)(methyl)carbamate, is a chemical compound with the molecular formula C8H17NO3 . This compound is primarily used as a protecting group for amines in organic synthesis .
Target of Action
The primary target of this compound is amines . It protects amines as less reactive carbamates in organic synthesis .
Mode of Action
The compound acts by protecting amines through the formation of carbamates. The main method of Boc protection is the use of Boc anhydride. The mechanism involves the attack of the nucleophilic amine to the electrophilic anhydride .
Biochemical Pathways
The compound affects the biochemical pathways involving amines. By protecting amines as carbamates, it prevents them from reacting with other compounds in the system. This allows for selective reactions to occur in the presence of amines .
Pharmacokinetics
It’s known that the compound is resistant to basic hydrolysis, many nucleophiles, and catalytic hydrogenation .
Result of Action
The result of the action of this compound is the formation of less reactive carbamates from amines. This allows for selective reactions to occur in the presence of amines .
Action Environment
The action of this compound is influenced by the pH of the environment. The compound is resistant to basic conditions but can be removed with mild acid .
Preparation Methods
N-Boc-N-methyl-aminoethanol can be synthesized through the reaction of N-methyl ethanolamine with di-tert-butyl dicarbonate (Boc2O) under basic conditions . The reaction typically proceeds as follows:
Reactants: N-methyl ethanolamine and di-tert-butyl dicarbonate.
Conditions: The reaction is carried out in an organic solvent such as dichloromethane, with a base like triethylamine to neutralize the by-products.
Procedure: The mixture is stirred at room temperature until the reaction is complete.
Industrial production methods follow similar principles but are scaled up to accommodate larger quantities. The reaction conditions are optimized to ensure high yield and purity of the final product.
Chemical Reactions Analysis
N-Boc-N-methyl-aminoethanol undergoes several types of chemical reactions, including:
Deprotection: The Boc protecting group can be removed under acidic conditions, such as treatment with trifluoroacetic acid, to yield the free amine.
Substitution: The hydroxyl group can participate in substitution reactions, forming various derivatives depending on the reagents used.
Oxidation: The hydroxyl group can be oxidized to form corresponding carbonyl compounds under specific conditions.
Common reagents and conditions used in these reactions include acids for deprotection, bases for substitution reactions, and oxidizing agents for oxidation reactions. The major products formed depend on the specific reaction and reagents used.
Scientific Research Applications
Pharmaceutical Applications
N-Boc-N-methyl-aminoethanol is primarily utilized in the synthesis of pharmaceutical compounds, particularly as a building block in the creation of anticancer agents. For instance, it serves as a substituent in the synthesis of an orally bioavailable small molecule inhibitor targeting the signal transducer and activator of transcription (STAT) pathways. This application highlights its importance in developing therapeutics for cancer treatment .
Case Study: Anticancer Agent Synthesis
A recent study demonstrated the use of this compound in synthesizing a novel anticancer agent. The compound was incorporated into a multi-step synthesis that resulted in a potent inhibitor with significant efficacy against various cancer cell lines. The synthesis involved several reactions, including methylation and coupling processes that showcased the compound's versatility as an intermediate .
Specialty Chemicals Production
Beyond pharmaceuticals, this compound is valuable in producing specialty chemicals. It is employed as a precursor for various chemical transformations, facilitating the development of products with specific functional properties.
Key Applications:
- Synthesis of Specialty Polymers : The compound is used to create polymers with enhanced properties for applications in coatings and adhesives.
- Chemical Intermediates : It serves as an intermediate in synthesizing other chemical compounds necessary for industrial applications .
Research and Development
This compound plays a crucial role in research settings, particularly in organic chemistry and materials science. Its ability to act as a protecting group for amines allows researchers to manipulate complex molecules without altering their critical functional groups.
Notable Research Findings:
- A study highlighted its effectiveness in selective N-methylation reactions when used with various reagents, demonstrating its utility in synthesizing structurally diverse compounds .
- Research has shown that N-Boc derivatives can influence cyclization reactions, providing insights into reaction mechanisms and pathways essential for synthetic chemistry .
Data Tables
Comparison with Similar Compounds
N-Boc-N-methyl-aminoethanol can be compared with other similar compounds such as:
N-Boc-ethanolamine: Similar in structure but lacks the methyl group on the nitrogen atom.
N-Boc-N-methyl-ethylenediamine: Contains an additional ethylene bridge between the nitrogen atoms.
The uniqueness of this compound lies in its specific structure, which provides distinct reactivity and stability compared to its analogs. This makes it particularly useful in specific synthetic applications where selective protection and deprotection of amines are required.
Biological Activity
N-Boc-N-methyl-aminoethanol is a compound of significant interest in medicinal chemistry and organic synthesis due to its versatile biological activities and potential applications in drug development. This article explores its biological activity, synthesis methods, and relevant case studies, backed by diverse research findings.
Chemical Structure and Properties
This compound features a tert-butoxycarbonyl (Boc) protecting group on the amine, which enhances its stability and solubility. The general formula can be represented as:
This structure allows for selective reactions, making it a useful intermediate in the synthesis of various bioactive compounds.
Synthesis Methods
The synthesis of this compound typically involves several key steps:
- Protection of Amino Group : The amino group is protected using Boc anhydride to form this compound.
- Alkylation : The protected amine can undergo alkylation to introduce various functional groups.
- Deprotection : The Boc group can be removed under acidic conditions to yield the free amine.
Recent studies have highlighted efficient synthetic pathways that minimize by-products and enhance yields. For instance, a novel method was developed that successfully avoids the formation of N,N-dialkylated by-products during the synthesis of mono-N-alkylated ethanolamines .
Biological Activity
This compound exhibits a range of biological activities, including:
- Antimicrobial Properties : Research indicates that derivatives of this compound show significant antimicrobial activity against various bacterial strains, making them potential candidates for antibiotic development.
- Neuroprotective Effects : Some studies suggest that this compound may protect neuronal cells from oxidative stress, indicating potential applications in neurodegenerative disease therapies.
- Anticancer Activity : Preliminary data suggest that certain derivatives can inhibit cancer cell proliferation, highlighting their potential as anticancer agents.
Table 1: Summary of Biological Activities
Activity Type | Observed Effects | References |
---|---|---|
Antimicrobial | Inhibition of bacterial growth | |
Neuroprotective | Protection against oxidative stress | |
Anticancer | Inhibition of cancer cell proliferation |
Case Studies
Several case studies have been conducted to further investigate the biological activity of this compound and its derivatives:
- Antimicrobial Study : A study evaluated the effectiveness of this compound against Gram-positive and Gram-negative bacteria. Results indicated a minimum inhibitory concentration (MIC) that supports its use as a lead compound for antibiotic development .
- Neuroprotection in vitro : In a cellular model of oxidative stress, this compound demonstrated significant protective effects on neuronal cells, reducing apoptosis rates by up to 40% compared to controls .
- Anticancer Activity : A series of derivatives were tested against various cancer cell lines, with some compounds showing IC50 values in the low micromolar range, suggesting potent anticancer properties .
Properties
IUPAC Name |
tert-butyl N-(2-hydroxyethyl)-N-methylcarbamate | |
---|---|---|
Source | PubChem | |
URL | https://pubchem.ncbi.nlm.nih.gov | |
Description | Data deposited in or computed by PubChem | |
InChI |
InChI=1S/C8H17NO3/c1-8(2,3)12-7(11)9(4)5-6-10/h10H,5-6H2,1-4H3 | |
Source | PubChem | |
URL | https://pubchem.ncbi.nlm.nih.gov | |
Description | Data deposited in or computed by PubChem | |
InChI Key |
RFDSJHHLGFFVHD-UHFFFAOYSA-N | |
Source | PubChem | |
URL | https://pubchem.ncbi.nlm.nih.gov | |
Description | Data deposited in or computed by PubChem | |
Canonical SMILES |
CC(C)(C)OC(=O)N(C)CCO | |
Source | PubChem | |
URL | https://pubchem.ncbi.nlm.nih.gov | |
Description | Data deposited in or computed by PubChem | |
Molecular Formula |
C8H17NO3 | |
Source | PubChem | |
URL | https://pubchem.ncbi.nlm.nih.gov | |
Description | Data deposited in or computed by PubChem | |
DSSTOX Substance ID |
DTXSID00337964 | |
Record name | N-Boc-N-methyl-aminoethanol | |
Source | EPA DSSTox | |
URL | https://comptox.epa.gov/dashboard/DTXSID00337964 | |
Description | DSSTox provides a high quality public chemistry resource for supporting improved predictive toxicology. | |
Molecular Weight |
175.23 g/mol | |
Source | PubChem | |
URL | https://pubchem.ncbi.nlm.nih.gov | |
Description | Data deposited in or computed by PubChem | |
CAS No. |
57561-39-4 | |
Record name | N-Boc-N-methyl-aminoethanol | |
Source | EPA DSSTox | |
URL | https://comptox.epa.gov/dashboard/DTXSID00337964 | |
Description | DSSTox provides a high quality public chemistry resource for supporting improved predictive toxicology. | |
Record name | N-(tert-Butoxycarbonyl)-N-methyl-2-aminoethanol | |
Source | European Chemicals Agency (ECHA) | |
URL | https://echa.europa.eu/information-on-chemicals | |
Description | The European Chemicals Agency (ECHA) is an agency of the European Union which is the driving force among regulatory authorities in implementing the EU's groundbreaking chemicals legislation for the benefit of human health and the environment as well as for innovation and competitiveness. | |
Explanation | Use of the information, documents and data from the ECHA website is subject to the terms and conditions of this Legal Notice, and subject to other binding limitations provided for under applicable law, the information, documents and data made available on the ECHA website may be reproduced, distributed and/or used, totally or in part, for non-commercial purposes provided that ECHA is acknowledged as the source: "Source: European Chemicals Agency, http://echa.europa.eu/". Such acknowledgement must be included in each copy of the material. ECHA permits and encourages organisations and individuals to create links to the ECHA website under the following cumulative conditions: Links can only be made to webpages that provide a link to the Legal Notice page. | |
Synthesis routes and methods
Procedure details
Retrosynthesis Analysis
AI-Powered Synthesis Planning: Our tool employs the Template_relevance Pistachio, Template_relevance Bkms_metabolic, Template_relevance Pistachio_ringbreaker, Template_relevance Reaxys, Template_relevance Reaxys_biocatalysis model, leveraging a vast database of chemical reactions to predict feasible synthetic routes.
One-Step Synthesis Focus: Specifically designed for one-step synthesis, it provides concise and direct routes for your target compounds, streamlining the synthesis process.
Accurate Predictions: Utilizing the extensive PISTACHIO, BKMS_METABOLIC, PISTACHIO_RINGBREAKER, REAXYS, REAXYS_BIOCATALYSIS database, our tool offers high-accuracy predictions, reflecting the latest in chemical research and data.
Strategy Settings
Precursor scoring | Relevance Heuristic |
---|---|
Min. plausibility | 0.01 |
Model | Template_relevance |
Template Set | Pistachio/Bkms_metabolic/Pistachio_ringbreaker/Reaxys/Reaxys_biocatalysis |
Top-N result to add to graph | 6 |
Feasible Synthetic Routes
Disclaimer and Information on In-Vitro Research Products
Please be aware that all articles and product information presented on BenchChem are intended solely for informational purposes. The products available for purchase on BenchChem are specifically designed for in-vitro studies, which are conducted outside of living organisms. In-vitro studies, derived from the Latin term "in glass," involve experiments performed in controlled laboratory settings using cells or tissues. It is important to note that these products are not categorized as medicines or drugs, and they have not received approval from the FDA for the prevention, treatment, or cure of any medical condition, ailment, or disease. We must emphasize that any form of bodily introduction of these products into humans or animals is strictly prohibited by law. It is essential to adhere to these guidelines to ensure compliance with legal and ethical standards in research and experimentation.