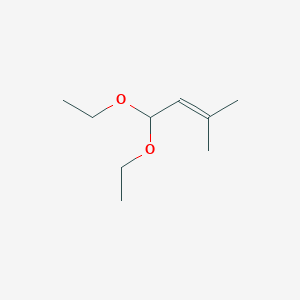
1,1-Diethoxy-3-methyl-2-butene
Overview
Description
1,1-Diethoxy-3-methyl-2-butene is an acyclic alkene with the molecular formula C9H18O2 and a molecular weight of 158.24 g/mol . It is a colorless liquid with a density of 0.837 g/mL at 25°C and a boiling point of 53-58°C at 20 mmHg . This compound is used in various chemical syntheses and has applications in different fields of scientific research.
Biochemical Analysis
Biochemical Properties
1,1-Diethoxy-3-methyl-2-butene plays a significant role in biochemical reactions, particularly in the synthesis of complex organic molecules. It interacts with enzymes, proteins, and other biomolecules to facilitate these reactions. For instance, it is used in the synthesis of angular pyranocoumarins and 6-cyano-2,2-dimethyl-2-H-1-benzopyran . The nature of these interactions often involves the formation of covalent bonds with the active sites of enzymes, leading to the formation of new chemical products.
Cellular Effects
The effects of this compound on various types of cells and cellular processes are profound. This compound influences cell function by affecting cell signaling pathways, gene expression, and cellular metabolism. For example, it can modulate the activity of certain enzymes involved in metabolic pathways, thereby altering the metabolic flux and levels of metabolites within the cell . These changes can have downstream effects on cellular processes such as growth, differentiation, and apoptosis.
Molecular Mechanism
At the molecular level, this compound exerts its effects through specific binding interactions with biomolecules. It can act as an enzyme inhibitor or activator, depending on the context of the reaction. The compound’s structure allows it to fit into the active sites of enzymes, where it can either block substrate binding or enhance the enzyme’s catalytic activity . Additionally, this compound can influence gene expression by interacting with transcription factors and other regulatory proteins.
Temporal Effects in Laboratory Settings
In laboratory settings, the effects of this compound can change over time. The compound’s stability and degradation are important factors to consider. It is known to be relatively stable under standard laboratory conditions, but it can undergo degradation when exposed to extreme temperatures or reactive chemicals . Long-term studies have shown that prolonged exposure to this compound can lead to changes in cellular function, including alterations in metabolic activity and gene expression.
Dosage Effects in Animal Models
The effects of this compound vary with different dosages in animal models. At low doses, the compound may have minimal impact on cellular function, while higher doses can lead to significant changes in metabolic pathways and enzyme activity . Toxic or adverse effects have been observed at very high doses, including cellular damage and disruption of normal physiological processes. These threshold effects highlight the importance of careful dosage control in experimental settings.
Metabolic Pathways
This compound is involved in several metabolic pathways. It interacts with enzymes and cofactors to facilitate the conversion of substrates into products. For example, it is used in the synthesis of 6,6,10-trimethyl-4-propyl-10,11-dihydro-2H,6H,12H-dipyrano[2,3-f:2′,3′-h]chromene-2,12-dione . These interactions can affect the overall metabolic flux and levels of specific metabolites within the cell.
Transport and Distribution
Within cells and tissues, this compound is transported and distributed through various mechanisms. It can interact with transporters and binding proteins that facilitate its movement across cellular membranes . The compound’s localization and accumulation within specific cellular compartments can influence its activity and function.
Subcellular Localization
The subcellular localization of this compound is crucial for its activity. It can be directed to specific compartments or organelles through targeting signals or post-translational modifications . These localization mechanisms ensure that the compound exerts its effects in the appropriate cellular context, thereby enhancing its efficacy in biochemical reactions.
Preparation Methods
1,1-Diethoxy-3-methyl-2-butene can be synthesized through the esterification reaction of 2-methyl-1,3-butadiene with ethanol . The reaction typically occurs under acidic conditions, using catalysts such as sulfuric acid, p-toluenesulfonic acid, or hydrochloric acid . The reaction conditions include maintaining a temperature of around 20°C and using p-xylene as a solvent .
Chemical Reactions Analysis
1,1-Diethoxy-3-methyl-2-butene undergoes various chemical reactions, including:
Oxidation: This compound can be oxidized to form corresponding aldehydes or carboxylic acids using oxidizing agents like potassium permanganate or chromium trioxide.
Reduction: It can be reduced to form alcohols using reducing agents such as lithium aluminum hydride or sodium borohydride.
Substitution: The compound can undergo nucleophilic substitution reactions, where the ethoxy groups can be replaced by other nucleophiles like halides or amines.
Scientific Research Applications
1,1-Diethoxy-3-methyl-2-butene is used in the synthesis of various organic compounds, including:
Mechanism of Action
The mechanism of action of 1,1-Diethoxy-3-methyl-2-butene involves its reactivity as an alkene. The double bond in the compound allows it to participate in various addition and substitution reactions. The ethoxy groups can act as leaving groups in nucleophilic substitution reactions, facilitating the formation of new compounds. The compound’s reactivity is influenced by the presence of the electron-donating ethoxy groups, which can stabilize reaction intermediates .
Comparison with Similar Compounds
1,1-Diethoxy-3-methyl-2-butene can be compared with other similar compounds such as:
2,3-Dimethyl-2-butene: This compound has a similar structure but lacks the ethoxy groups, making it less reactive in nucleophilic substitution reactions.
2-Methyl-2-butene: This compound also lacks the ethoxy groups and has different reactivity patterns compared to this compound.
3-Methyl-1-butene: This compound has a different position of the double bond and lacks the ethoxy groups, resulting in different chemical properties.
This compound is unique due to the presence of the ethoxy groups, which enhance its reactivity and make it suitable for various synthetic applications.
Properties
IUPAC Name |
1,1-diethoxy-3-methylbut-2-ene | |
---|---|---|
Source | PubChem | |
URL | https://pubchem.ncbi.nlm.nih.gov | |
Description | Data deposited in or computed by PubChem | |
InChI |
InChI=1S/C9H18O2/c1-5-10-9(11-6-2)7-8(3)4/h7,9H,5-6H2,1-4H3 | |
Source | PubChem | |
URL | https://pubchem.ncbi.nlm.nih.gov | |
Description | Data deposited in or computed by PubChem | |
InChI Key |
SFUKGEREDDIOED-UHFFFAOYSA-N | |
Source | PubChem | |
URL | https://pubchem.ncbi.nlm.nih.gov | |
Description | Data deposited in or computed by PubChem | |
Canonical SMILES |
CCOC(C=C(C)C)OCC | |
Source | PubChem | |
URL | https://pubchem.ncbi.nlm.nih.gov | |
Description | Data deposited in or computed by PubChem | |
Molecular Formula |
C9H18O2 | |
Source | PubChem | |
URL | https://pubchem.ncbi.nlm.nih.gov | |
Description | Data deposited in or computed by PubChem | |
DSSTOX Substance ID |
DTXSID00410564 | |
Record name | 1,1-Diethoxy-3-methyl-2-butene | |
Source | EPA DSSTox | |
URL | https://comptox.epa.gov/dashboard/DTXSID00410564 | |
Description | DSSTox provides a high quality public chemistry resource for supporting improved predictive toxicology. | |
Molecular Weight |
158.24 g/mol | |
Source | PubChem | |
URL | https://pubchem.ncbi.nlm.nih.gov | |
Description | Data deposited in or computed by PubChem | |
CAS No. |
1740-74-5 | |
Record name | 1,1-Diethoxy-3-methyl-2-butene | |
Source | EPA DSSTox | |
URL | https://comptox.epa.gov/dashboard/DTXSID00410564 | |
Description | DSSTox provides a high quality public chemistry resource for supporting improved predictive toxicology. | |
Record name | 1,1-Diethoxy-3-methyl-2-butene | |
Source | European Chemicals Agency (ECHA) | |
URL | https://echa.europa.eu/information-on-chemicals | |
Description | The European Chemicals Agency (ECHA) is an agency of the European Union which is the driving force among regulatory authorities in implementing the EU's groundbreaking chemicals legislation for the benefit of human health and the environment as well as for innovation and competitiveness. | |
Explanation | Use of the information, documents and data from the ECHA website is subject to the terms and conditions of this Legal Notice, and subject to other binding limitations provided for under applicable law, the information, documents and data made available on the ECHA website may be reproduced, distributed and/or used, totally or in part, for non-commercial purposes provided that ECHA is acknowledged as the source: "Source: European Chemicals Agency, http://echa.europa.eu/". Such acknowledgement must be included in each copy of the material. ECHA permits and encourages organisations and individuals to create links to the ECHA website under the following cumulative conditions: Links can only be made to webpages that provide a link to the Legal Notice page. | |
Synthesis routes and methods I
Procedure details
Synthesis routes and methods II
Procedure details
Synthesis routes and methods III
Procedure details
Retrosynthesis Analysis
AI-Powered Synthesis Planning: Our tool employs the Template_relevance Pistachio, Template_relevance Bkms_metabolic, Template_relevance Pistachio_ringbreaker, Template_relevance Reaxys, Template_relevance Reaxys_biocatalysis model, leveraging a vast database of chemical reactions to predict feasible synthetic routes.
One-Step Synthesis Focus: Specifically designed for one-step synthesis, it provides concise and direct routes for your target compounds, streamlining the synthesis process.
Accurate Predictions: Utilizing the extensive PISTACHIO, BKMS_METABOLIC, PISTACHIO_RINGBREAKER, REAXYS, REAXYS_BIOCATALYSIS database, our tool offers high-accuracy predictions, reflecting the latest in chemical research and data.
Strategy Settings
Precursor scoring | Relevance Heuristic |
---|---|
Min. plausibility | 0.01 |
Model | Template_relevance |
Template Set | Pistachio/Bkms_metabolic/Pistachio_ringbreaker/Reaxys/Reaxys_biocatalysis |
Top-N result to add to graph | 6 |
Feasible Synthetic Routes
Disclaimer and Information on In-Vitro Research Products
Please be aware that all articles and product information presented on BenchChem are intended solely for informational purposes. The products available for purchase on BenchChem are specifically designed for in-vitro studies, which are conducted outside of living organisms. In-vitro studies, derived from the Latin term "in glass," involve experiments performed in controlled laboratory settings using cells or tissues. It is important to note that these products are not categorized as medicines or drugs, and they have not received approval from the FDA for the prevention, treatment, or cure of any medical condition, ailment, or disease. We must emphasize that any form of bodily introduction of these products into humans or animals is strictly prohibited by law. It is essential to adhere to these guidelines to ensure compliance with legal and ethical standards in research and experimentation.