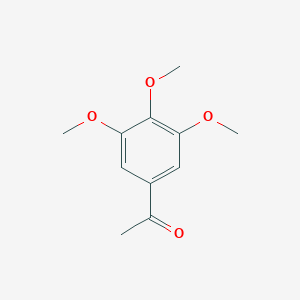
3',4',5'-Trimethoxyacetophenone
Overview
Description
3',4',5'-Trimethoxyacetophenone is a chemical compound that is structurally related to acetophenone derivatives with methoxy groups attached to the aromatic ring. These compounds are of interest due to their presence in various natural products and their potential biological activities.
Synthesis Analysis
The synthesis of related compounds involves multi-step reactions, often starting from simpler acetophenone derivatives or related aromatic compounds. For instance, the synthesis of 2-hydroxy-4,5,6-trimethoxyacetophenone was achieved through a 5-step reaction sequence starting from trimethoxybenzene, which included oxidation, reduction, Friedel-Crafts acylation, hydrolysis, and methylation, with a yield of 48% . Similarly, 3,4,5-trimethoxyphenyllithium, a related compound, was synthesized from its bromide or iodide form and used for subsequent addition reactions to introduce the trimethoxyphenyl group via carbon-carbon bond formation .
Molecular Structure Analysis
The molecular structure of these compounds is characterized using techniques such as Nuclear Magnetic Resonance (NMR) and single crystal X-ray diffraction. For example, the structure of 2-methoxy-5-(2',3',4'-trimethoxyphenyl)tropone was determined using X-ray diffraction, revealing a conformation similar to colchicine despite the absence of a middle ring . The crystal structure of 2-hydroxy-3,4,6-trimethoxyacetophenone was also determined at different temperatures, and its vibrational properties were characterized through spectroscopy .
Chemical Reactions Analysis
The reactivity of these compounds can be inferred from their functional groups and electronic structure. The presence of methoxy groups can influence the electronic distribution and reactivity of the aromatic ring. For instance, the electrophilic substitution reactions might be facilitated by the electron-donating effect of the methoxy groups. The synthesis of 3,4,5-trimethoxyphenyllithium suggests that the trimethoxyphenyl group can be introduced into various molecules through carbon-carbon bond-forming reactions .
Physical and Chemical Properties Analysis
The physical and chemical properties of these compounds are closely related to their molecular structure. The vibrational wavenumbers, hyperpolarizability, and infrared intensities of these molecules can be computed using computational methods such as Hartree-Fock (HF) and Density Functional Theory (DFT) . The HOMO and LUMO analysis, as well as the Molecular Electrostatic Potential (MEP) map, provide insights into the charge transfer within the molecule and the sites of chemical reactivity . The intermolecular interactions within the crystal structures can be analyzed using Hirshfeld surface analysis, which helps to understand the stability and packing of the molecules in the solid state .
Scientific Research Applications
Kinetic Studies in Organic Synthesis
3',4',5'-Trimethoxyacetophenone has been utilized in organic synthesis. For instance, it was used to study the kinetic isotope effect in ortho-metallation processes, demonstrating its role in understanding reaction mechanisms in organic chemistry (Gommans, Main, & Nicholson, 1986).
Structural Characterization and Antimicrobial Potential
In another study, 2-Hydroxy-3,4,6-trimethoxyacetophenone, a closely related compound, was isolated and its structure characterized using various spectroscopic methods. This compound showed potential antimicrobial activity (Santiago et al., 2018).
Synthesis of Intermediate Compounds
The synthesis of 2-hydroxy-4,5,6-trimethoxyacetophenone, a related compound, was achieved through a multi-step process, highlighting its importance in the synthesis of intermediate compounds in chemical reactions (Tu Wei-jun, 2013).
Photocleavage and Photostabilization Studies
Research has also been conducted on the photocleavage of 3,4,5-trimethoxy-α-(2′,6′-dimethoxyphenoxy)-acetophenone and its implications in the photostabilization of mechanical pulps, showing its application in material sciences (Harvey & Ragauskas, 1996).
Study of Electrophilic Displacement
The electrophilic displacement reactions of 2,4,6-trimethoxyacetophenone have been explored, providing insights into the reactivity of such compounds in chemical synthesis (Strating, Thijs, & Zwanenburg, 2010).
Antimicrobial Activity of Derivatives
Chalcones synthesized from 2-hydroxy-3,4,6-trimethoxyacetophenone demonstrated antibacterial activity, suggesting the potential of its derivatives in drug development (Freitas et al., 2020).
Spectral Properties in Inorganic Chemistry
The influence of restricted rotation on the spectral properties of acetophenonetricarbonylchromium complexes, including 3,4,5-trimethoxyacetophenone derivatives, was investigated, showcasing its use in inorganic chemistry and material sciences (Armstrong et al., 1990).
Antioxidant Studies
In the study of antioxidants, derivatives of 2,4,5,7,8-pentamethyl-4H-1,3-benzodioxin-6-ol, obtained from compounds like 2,4,6-trimethoxyacetophenone, were synthesized and examined for their antioxidant properties (Rosenau et al., 2002).
Safety and Hazards
properties
IUPAC Name |
1-(3,4,5-trimethoxyphenyl)ethanone | |
---|---|---|
Source | PubChem | |
URL | https://pubchem.ncbi.nlm.nih.gov | |
Description | Data deposited in or computed by PubChem | |
InChI |
InChI=1S/C11H14O4/c1-7(12)8-5-9(13-2)11(15-4)10(6-8)14-3/h5-6H,1-4H3 | |
Source | PubChem | |
URL | https://pubchem.ncbi.nlm.nih.gov | |
Description | Data deposited in or computed by PubChem | |
InChI Key |
VUGQIIQFXCXZJU-UHFFFAOYSA-N | |
Source | PubChem | |
URL | https://pubchem.ncbi.nlm.nih.gov | |
Description | Data deposited in or computed by PubChem | |
Canonical SMILES |
CC(=O)C1=CC(=C(C(=C1)OC)OC)OC | |
Source | PubChem | |
URL | https://pubchem.ncbi.nlm.nih.gov | |
Description | Data deposited in or computed by PubChem | |
Molecular Formula |
C11H14O4 | |
Source | PubChem | |
URL | https://pubchem.ncbi.nlm.nih.gov | |
Description | Data deposited in or computed by PubChem | |
DSSTOX Substance ID |
DTXSID30150481 | |
Record name | 3',4',5'-Trimethoxyacetophenone | |
Source | EPA DSSTox | |
URL | https://comptox.epa.gov/dashboard/DTXSID30150481 | |
Description | DSSTox provides a high quality public chemistry resource for supporting improved predictive toxicology. | |
Molecular Weight |
210.23 g/mol | |
Source | PubChem | |
URL | https://pubchem.ncbi.nlm.nih.gov | |
Description | Data deposited in or computed by PubChem | |
Product Name |
3',4',5'-Trimethoxyacetophenone | |
CAS RN |
1136-86-3 | |
Record name | 3′,4′,5′-Trimethoxyacetophenone | |
Source | CAS Common Chemistry | |
URL | https://commonchemistry.cas.org/detail?cas_rn=1136-86-3 | |
Description | CAS Common Chemistry is an open community resource for accessing chemical information. Nearly 500,000 chemical substances from CAS REGISTRY cover areas of community interest, including common and frequently regulated chemicals, and those relevant to high school and undergraduate chemistry classes. This chemical information, curated by our expert scientists, is provided in alignment with our mission as a division of the American Chemical Society. | |
Explanation | The data from CAS Common Chemistry is provided under a CC-BY-NC 4.0 license, unless otherwise stated. | |
Record name | 3',4',5'-Trimethoxyacetophenone | |
Source | ChemIDplus | |
URL | https://pubchem.ncbi.nlm.nih.gov/substance/?source=chemidplus&sourceid=0001136863 | |
Description | ChemIDplus is a free, web search system that provides access to the structure and nomenclature authority files used for the identification of chemical substances cited in National Library of Medicine (NLM) databases, including the TOXNET system. | |
Record name | 1136-86-3 | |
Source | DTP/NCI | |
URL | https://dtp.cancer.gov/dtpstandard/servlet/dwindex?searchtype=NSC&outputformat=html&searchlist=30099 | |
Description | The NCI Development Therapeutics Program (DTP) provides services and resources to the academic and private-sector research communities worldwide to facilitate the discovery and development of new cancer therapeutic agents. | |
Explanation | Unless otherwise indicated, all text within NCI products is free of copyright and may be reused without our permission. Credit the National Cancer Institute as the source. | |
Record name | 3',4',5'-Trimethoxyacetophenone | |
Source | EPA DSSTox | |
URL | https://comptox.epa.gov/dashboard/DTXSID30150481 | |
Description | DSSTox provides a high quality public chemistry resource for supporting improved predictive toxicology. | |
Record name | 3',4',5'-trimethoxyacetophenone | |
Source | European Chemicals Agency (ECHA) | |
URL | https://echa.europa.eu/substance-information/-/substanceinfo/100.013.183 | |
Description | The European Chemicals Agency (ECHA) is an agency of the European Union which is the driving force among regulatory authorities in implementing the EU's groundbreaking chemicals legislation for the benefit of human health and the environment as well as for innovation and competitiveness. | |
Explanation | Use of the information, documents and data from the ECHA website is subject to the terms and conditions of this Legal Notice, and subject to other binding limitations provided for under applicable law, the information, documents and data made available on the ECHA website may be reproduced, distributed and/or used, totally or in part, for non-commercial purposes provided that ECHA is acknowledged as the source: "Source: European Chemicals Agency, http://echa.europa.eu/". Such acknowledgement must be included in each copy of the material. ECHA permits and encourages organisations and individuals to create links to the ECHA website under the following cumulative conditions: Links can only be made to webpages that provide a link to the Legal Notice page. | |
Record name | 3',4',5'-TRIMETHOXYACETOPHENONE | |
Source | FDA Global Substance Registration System (GSRS) | |
URL | https://gsrs.ncats.nih.gov/ginas/app/beta/substances/35KU75558G | |
Description | The FDA Global Substance Registration System (GSRS) enables the efficient and accurate exchange of information on what substances are in regulated products. Instead of relying on names, which vary across regulatory domains, countries, and regions, the GSRS knowledge base makes it possible for substances to be defined by standardized, scientific descriptions. | |
Explanation | Unless otherwise noted, the contents of the FDA website (www.fda.gov), both text and graphics, are not copyrighted. They are in the public domain and may be republished, reprinted and otherwise used freely by anyone without the need to obtain permission from FDA. Credit to the U.S. Food and Drug Administration as the source is appreciated but not required. | |
Retrosynthesis Analysis
AI-Powered Synthesis Planning: Our tool employs the Template_relevance Pistachio, Template_relevance Bkms_metabolic, Template_relevance Pistachio_ringbreaker, Template_relevance Reaxys, Template_relevance Reaxys_biocatalysis model, leveraging a vast database of chemical reactions to predict feasible synthetic routes.
One-Step Synthesis Focus: Specifically designed for one-step synthesis, it provides concise and direct routes for your target compounds, streamlining the synthesis process.
Accurate Predictions: Utilizing the extensive PISTACHIO, BKMS_METABOLIC, PISTACHIO_RINGBREAKER, REAXYS, REAXYS_BIOCATALYSIS database, our tool offers high-accuracy predictions, reflecting the latest in chemical research and data.
Strategy Settings
Precursor scoring | Relevance Heuristic |
---|---|
Min. plausibility | 0.01 |
Model | Template_relevance |
Template Set | Pistachio/Bkms_metabolic/Pistachio_ringbreaker/Reaxys/Reaxys_biocatalysis |
Top-N result to add to graph | 6 |
Feasible Synthetic Routes
Q & A
Q1: What is the mechanism by which 3',4',5'-Trimethoxyacetophenone interacts with tubulin and what are the downstream effects?
A1: 3',4',5'-Trimethoxyacetophenone acts as a fast-binding analog of the colchicine A-ring, competing for the colchicine binding site on tubulin. [] While the exact mechanism is not fully elucidated, studies indicate that the A-ring of colchicine analogs, including 3',4',5'-Trimethoxyacetophenone, plays a crucial role in the initial binding step to tubulin. [, ] This binding interferes with tubulin polymerization, a process essential for microtubule formation. Microtubules are crucial for cell division, and disruption of their formation leads to mitotic arrest, ultimately impacting cell viability.
Q2: Can you elaborate on the role of the A-ring in the binding of colchicine and its analogs to tubulin based on the provided research?
A2: Research suggests that both the A and C rings of colchicine analogs are involved in binding to tubulin. [] Specifically, kinetic studies using 2-Methoxy-5-(2',4'-dimethoxyphenyl)-2,4,6-cycloheptatrien-1-one (MDC), a colchicine analog with a modified A-ring, show significant differences in the binding pathway compared to colchicine and its close analog MTC. [] This difference, attributed to the A-ring modification in MDC, highlights the importance of this structural feature in the initial binding step. Furthermore, the presence of 3',4',5'-Trimethoxyacetophenone, mimicking the A-ring structure, slows down the binding kinetics of other colchicine analogs, supporting the competitive nature of interaction at the A-ring binding subsite on tubulin. []
Q3: Could you provide the molecular formula, weight, and key spectroscopic data for 3',4',5'-Trimethoxyacetophenone?
A3:
Q4: Are there any insights from the research regarding the Structure-Activity Relationship (SAR) of 3',4',5'-Trimethoxyacetophenone or related compounds?
A4: While the provided research focuses primarily on the interaction with tubulin, one study explores the SAR of chalcone derivatives as AMPK activators. [] Although not directly related to 3',4',5'-Trimethoxyacetophenone, this research highlights the importance of specific substitutions on both the A and B rings of chalcones for AMPK activity. For instance, a 3-methoxy group on the B-ring and 3,4-disubstitutions were found to be beneficial for activity. This emphasizes the potential impact of even minor structural modifications on the biological activity of aromatic compounds. Further research specifically targeting the SAR of 3',4',5'-Trimethoxyacetophenone analogs could provide valuable insights for developing compounds with improved potency and selectivity towards specific targets.
Disclaimer and Information on In-Vitro Research Products
Please be aware that all articles and product information presented on BenchChem are intended solely for informational purposes. The products available for purchase on BenchChem are specifically designed for in-vitro studies, which are conducted outside of living organisms. In-vitro studies, derived from the Latin term "in glass," involve experiments performed in controlled laboratory settings using cells or tissues. It is important to note that these products are not categorized as medicines or drugs, and they have not received approval from the FDA for the prevention, treatment, or cure of any medical condition, ailment, or disease. We must emphasize that any form of bodily introduction of these products into humans or animals is strictly prohibited by law. It is essential to adhere to these guidelines to ensure compliance with legal and ethical standards in research and experimentation.