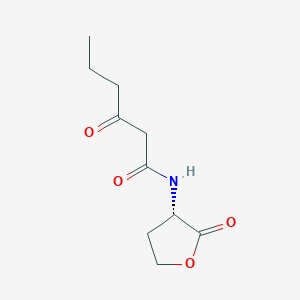
N-(3-Oxohexanoyl)-L-homoserine lactone
Overview
Description
N-(3-Oxohexanoyl)-L-homoserine lactone (C₁₀H₁₅NO₄; molecular weight 213.233) is a key acyl-homoserine lactone (AHL) quorum-sensing (QS) signal molecule. It features a six-carbon acyl chain with a 3-oxo substitution and a homoserine lactone ring . This compound is notable for its role in bacterial communication, particularly in Vibrio fischeri, where it regulates bioluminescence via the lux operon . It also acts as an autoregulator of carbapenem antibiotic biosynthesis in Erwinia carotovora and modulates virulence in plant pathogens like Pseudomonas syringae .
Preparation Methods
Synthetic Routes and Reaction Conditions: The synthesis of N-(3-Oxohexanoyl)-L-homoserine lactone typically involves the condensation of 3-oxohexanoic acid with L-homoserine lactone. This reaction is usually carried out in the presence of a coupling agent such as dicyclohexylcarbodiimide (DCC) and a catalyst like 4-dimethylaminopyridine (DMAP) under anhydrous conditions .
Industrial Production Methods: Industrial production of this compound can be achieved through microbial fermentation. Specific bacterial strains, such as Agrobacterium tumefaciens, are engineered to overproduce this compound. The fermentation process is optimized by controlling parameters such as temperature, pH, and nutrient availability to maximize yield .
Chemical Reactions Analysis
Types of Reactions: N-(3-Oxohexanoyl)-L-homoserine lactone undergoes various chemical reactions, including:
Hydrolysis: The lactone ring can be hydrolyzed under acidic or basic conditions to yield the corresponding carboxylic acid and amino alcohol.
Oxidation: The compound can be oxidized to form more oxidized derivatives, which may affect its signaling properties.
Substitution: The acyl chain can undergo substitution reactions, leading to the formation of different N-acyl homoserine lactones.
Common Reagents and Conditions:
Hydrolysis: Acidic or basic aqueous solutions.
Oxidation: Oxidizing agents such as potassium permanganate or hydrogen peroxide.
Substitution: Various alkylating agents under controlled conditions.
Major Products:
Hydrolysis Products: 3-oxohexanoic acid and L-homoserine.
Oxidation Products: Oxidized derivatives of the original compound.
Substitution Products: Different N-acyl homoserine lactones with modified acyl chains.
Scientific Research Applications
Quorum Sensing and Gene Regulation
Quorum Sensing Mechanism
3OC6-HSL plays a pivotal role in quorum sensing, a process where bacteria communicate through chemical signals to coordinate behavior based on population density. In the marine bacterium Vibrio fischeri, 3OC6-HSL binds to the LuxR protein, forming a transcriptional activation complex that induces the expression of bioluminescence genes (luxICDABEG operon) . This mechanism exemplifies how AHLs can regulate gene expression in response to environmental cues.
Case Study: Bioluminescence in Vibrio fischeri
In a study examining the quantification of 3OC6-HSL, researchers developed a bioluminescent strain of Escherichia coli as a bioreporter to measure 3OC6-HSL concentrations. This approach highlighted the compound's role in inducing bioluminescence and provided a method for rapid quantification in various supernatant backgrounds .
Regulation of Virulence
Virulence Factor Modulation
3OC6-HSL is implicated in regulating virulence factors in several pathogenic bacteria. For instance, it has been shown to influence the production of virulence factors in Erwinia carotovora, where it acts as an autoregulator for carbapenem antibiotic biosynthesis. The L-isomer of 3OC6-HSL demonstrated greater activity than its D-isomer, indicating its significance in bacterial pathogenicity .
Case Study: Carbapenem Production
Research indicated that 3OC6-HSL regulates carbapenem production by influencing gene expression related to antibiotic synthesis. This regulation underscores the potential for targeting quorum sensing pathways to develop novel antimicrobial strategies .
Biofilm Formation
Biofilm Development
3OC6-HSL is also involved in biofilm formation, a critical factor in bacterial survival and pathogenicity. It has been observed that higher concentrations of this signaling molecule promote biofilm development in various bacterial species .
Implications for Infection Control
Understanding how 3OC6-HSL influences biofilm formation can inform strategies to disrupt these communities and prevent chronic infections associated with biofilms.
Therapeutic Applications
Immunomodulatory Effects
Emerging research suggests that AHLs like 3OC6-HSL may have immunomodulatory effects. For example, studies have shown that certain AHLs can inhibit lymphocyte proliferation and modulate cytokine production in macrophages . These findings open avenues for exploring AHLs as potential therapeutic agents in immune-related disorders.
Summary Table of Applications
Mechanism of Action
N-(3-Oxohexanoyl)-L-homoserine lactone exerts its effects through quorum sensing. It diffuses across bacterial cell membranes and binds to specific receptor proteins, such as LuxR-type transcriptional regulators. This binding activates the transcription of target genes involved in various bacterial processes, including virulence, biofilm formation, and secondary metabolite production. The molecular targets and pathways involved are highly specific to the bacterial species and the environmental context .
Comparison with Similar Compounds
Comparison with Structurally Similar AHLs
AHLs vary in acyl chain length (C4–C14), substituents (3-oxo, 3-hydroxy, or unmodified), and biological functions. Below is a systematic comparison:
Structural Features
AHL Compound | Acyl Chain Length | Substituent | Molecular Weight | Key Structural Differences vs. 3OC6-HSL |
---|---|---|---|---|
N-Butyryl-L-HSL (C4-HSL) | 4 | None | 171.20 | Shorter chain; lacks 3-oxo group |
N-Hexanoyl-L-HSL (C6-HSL) | 6 | None | 199.24 | Same chain length; lacks 3-oxo group |
N-(3-Oxohexanoyl)-L-HSL (3OC6-HSL) | 6 | 3-oxo | 213.23 | Reference compound |
N-(3-Oxododecanoyl)-L-HSL (3OC12-HSL) | 12 | 3-oxo | 297.40 | Longer chain; higher hydrophobicity |
N-(3-Hydroxyhexanoyl)-L-HSL | 6 | 3-hydroxy | 215.24 | 3-hydroxy instead of 3-oxo; altered polarity |
N-Butyryl-L-HSL (C4-HSL)
- Source: Pseudomonas aeruginosa .
- Function : Regulates biofilm formation and virulence factors.
- Key Difference : Shorter chains like C4-HSL are less stable in hydrophobic environments compared to 3OC6-HSL .
N-Hexanoyl-L-HSL (C6-HSL)
- Source : Pseudomonas aureofaciens .
- Function : Controls phenazine antibiotic production.
- Key Difference : The absence of a 3-oxo group reduces receptor-binding affinity in Erwinia carotovora compared to 3OC6-HSL .
N-(3-Oxododecanoyl)-L-HSL (3OC12-HSL)
- Source: Pseudomonas aeruginosa .
- Function : Induces apoptosis in mast cells and modulates immune responses.
- Key Difference : Longer chains (C12) enhance membrane permeability and cytotoxicity, unlike 3OC6-HSL .
N-(3-Hydroxyhexanoyl)-L-HSL
- Source : Pseudomonas fluorescens .
- Function: Unknown specific role; detected in environmental isolates.
Receptor Specificity and Signaling Efficiency
- 3OC6-HSL : Binds LuxR-type receptors (e.g., CarR in Erwinia carotovora) with a dissociation constant (Kd) of 1.8 µM, activating carbapenem synthesis at thresholds as low as 0.5 µg/mL .
- C6-HSL : Requires 10-fold higher concentrations to achieve similar effects in Erwinia, highlighting the critical role of the 3-oxo group .
- 3OC12-HSL: Interacts with LasR in P.
Degradation and Stability
- Enzymatic Degradation: Variovorax paradoxus metabolizes 3OC6-HSL and other AHLs, with growth yields proportional to acyl chain length . AiiASS6 lactonase from Bacillus subtilis preferentially degrades N-(3-oxooctanoyl)-L-HSL (3OC8-HSL) over 3OC6-HSL, indicating substrate specificity .
- Chemical Stability : 3-Oxo derivatives are more prone to alkaline hydrolysis than unsubstituted AHLs due to the electron-withdrawing ketone group .
Detection and Analytical Differentiation
- Thin-Layer Chromatography (TLC) : 3OC6-HSL migrates uniquely compared to 3-hydroxy or unmodified AHLs, enabling identification in bacterial extracts .
- Biosensors: Agrobacterium tumefaciens NTL4 (pZLR4) detects 3OC6-HSL at nanomolar concentrations, while Chromobacterium violaceum CV026 is insensitive to it .
Biological Activity
N-(3-Oxohexanoyl)-L-homoserine lactone (OHHL) is a significant signaling molecule involved in quorum sensing, particularly in Gram-negative bacteria. This article provides an in-depth examination of its biological activity, mechanisms of action, and implications for antibiotic production.
Overview of this compound
This compound is classified as an acyl-homoserine lactone (AHL), a group of molecules that play critical roles in bacterial communication. It is synthesized by various bacterial species and acts as an autoregulator for gene expression, particularly in the biosynthesis of antibiotics such as carbapenems.
- Quorum Sensing : OHHL is produced by bacteria in response to population density. As the concentration of OHHL increases, it binds to specific receptors (LuxR-type proteins) in the bacterial cells, leading to the activation of target genes associated with virulence and antibiotic production.
- Regulation of Antibiotic Production : In Erwinia carotovora, OHHL regulates the biosynthesis of carbapenem antibiotics. The binding affinity of OHHL to the CarR protein has been quantified, showing a dissociation constant (Kd) of approximately 1.8 µM, which aligns with the concentration needed for effective induction of carbapenem production .
Biological Activity and Effects
- Induction Threshold : The L-isomer of OHHL exhibits a lower induction threshold for carbapenem production compared to its D counterpart. Studies indicate that an effective concentration is around 0.5 µg/ml for inducing biosynthesis .
- Cross-Species Interaction : OHHL not only regulates its own species but can also influence other bacteria through cross-signaling mechanisms. For instance, it has been shown to restore antibiotic production in mutant strains that lack the ability to produce carbapenems independently .
Study 1: Regulation in Erwinia carotovora
A detailed investigation revealed that mutants unable to produce carbapenems could regain this ability when exposed to OHHL. The study employed solvent extraction and chromatography techniques to isolate and characterize OHHL from bacterial cultures, confirming its role as a signaling molecule essential for antibiotic production .
Study 2: Immunomodulatory Effects
Research on related AHLs, such as N-(3-oxododecanoyl)-L-homoserine lactone, demonstrated immunomodulatory effects in animal models. This suggests that OHHL may also play a role in modulating host immune responses during bacterial infections .
Data Table: Biological Activity Summary
Activity | Description |
---|---|
Molecular Structure | This compound |
Induction Threshold | ~0.5 µg/ml for L-isomer |
Binding Affinity (Kd) | 1.8 µM with CarR protein |
Role in Antibiotic Production | Autoregulates carbapenem biosynthesis |
Cross-Species Effects | Can restore antibiotic production in mutant strains |
Q & A
Basic Research Questions
Q. How is OHHL detected and quantified in bacterial cultures?
OHHL detection typically involves thin-layer chromatography (TLC) coupled with biosensor strains (e.g., Agrobacterium tumefaciens with a lacZ reporter) to visualize spots corresponding to AHLs. The mobility and spot shape on TLC help tentatively identify OHHL, which can be confirmed via mass spectrometry . Quantification is achieved by measuring β-galactosidase activity in biosensor strains, as signal intensity correlates with OHHL concentration . For improved accuracy, control pH (ideally <7.0) to minimize lactonolysis during extraction .
Q. What factors influence OHHL stability in experimental setups?
OHHL stability depends on pH, temperature, and acyl chain length. At neutral/alkaline pH, lactonolysis (ring-opening) occurs, accelerating degradation. At pH 2.0, the lactone ring re-forms, stabilizing the molecule . Higher temperatures (e.g., 37°C vs. 22°C) increase degradation rates. Long-term storage at -20°C in anhydrous solvents (e.g., chloroform) is recommended to preserve activity .
Q. What is OHHL’s role in bacterial quorum sensing (QS)?
OHHL acts as an autoinducer in QS systems, regulating virulence, antibiotic production (e.g., carbapenem in Erwinia carotovora), and biofilm formation. It binds LuxR-type transcriptional regulators, activating target genes at threshold concentrations . Cross-feeding assays with QS-deficient mutants can validate its role in specific pathways .
Advanced Research Questions
Q. How can contradictory data on OHHL bioactivity across studies be resolved?
Contradictions often arise from strain-specific AHL profiles or environmental factors. For example, Erwinia carotovora strain SCC1 produces OHHL as a major signal, while SCC3193 generates trace amounts . Validate findings using:
- Genetic knockout models : Disrupt luxI homologs to confirm OHHL dependency.
- Analytical chemistry : Use LC-MS/MS to quantify OHHL directly, avoiding biosensor variability .
- pH monitoring : Ensure lactonolysis is controlled during experiments .
Q. What methodologies identify microbial degradation of OHHL in complex environments?
OHHL degradation by bacteria (e.g., Rhodococcus erythropolis or Variovorax paradoxus) involves:
- Oxidoreductase activity : Converts 3-oxo-OHHL to 3-hydroxy derivatives, detectable via HPLC with UV/fluorescence .
- Amidolytic cleavage : Releases homoserine lactone, identified using dansyl chloride derivatization .
- Isotope tracing : Radiolabeled OHHL (e.g., ¹⁴C) tracks mineralization to CO₂ .
Q. How do acyl chain modifications impact OHHL’s receptor specificity and stability?
The 3-oxohexanoyl group enhances receptor binding affinity (e.g., LuxR in Vibrio fischeri) compared to unsubstituted chains. However, longer acyl chains (e.g., C12) increase stability due to reduced lactonolysis . To study structure-activity relationships:
- Synthesize analogs (e.g., 3-hydroxy or shorter chains) via acylation of L-homoserine lactone with modified carboxylic acids .
- Test bioactivity using reporter strains (e.g., E. coli with LuxR-PₗuxI-GFP) .
Q. Methodological Challenges and Solutions
Designing experiments to study OHHL in polymicrobial communities
- Co-culture assays : Use transwell systems to separate OHHL-producing and degrading species (e.g., Pseudomonas and Rhodococcus) .
- Metabolomic profiling : Combine SPE (solid-phase extraction) with high-resolution MS to distinguish OHHL from structurally similar AHLs .
Optimizing OHHL extraction from biofilms
- Solvent selection : Use ethyl acetate or dichloromethane for high recovery rates. Avoid aqueous phases to prevent lactonolysis .
- Biofilm disruption : Employ sonication or enzymatic lysis (e.g., DNase/protease) to release matrix-embedded OHHL .
Q. Data Interpretation Tables
Table 1. OHHL Stability Under Varying Conditions
Condition | Half-Life (h) | Key Observation | Reference |
---|---|---|---|
pH 7.0, 37°C | 12 | Rapid lactonolysis | |
pH 5.0, 37°C | 48 | Moderate degradation | |
pH 2.0, 4°C | >168 | Stable lactone form | |
Presence of V. paradoxus | <6 | Complete biodegradation |
Table 2. OHHL Bioactivity in Model Organisms
Q. Key Recommendations
Properties
IUPAC Name |
3-oxo-N-[(3S)-2-oxooxolan-3-yl]hexanamide | |
---|---|---|
Source | PubChem | |
URL | https://pubchem.ncbi.nlm.nih.gov | |
Description | Data deposited in or computed by PubChem | |
InChI |
InChI=1S/C10H15NO4/c1-2-3-7(12)6-9(13)11-8-4-5-15-10(8)14/h8H,2-6H2,1H3,(H,11,13)/t8-/m0/s1 | |
Source | PubChem | |
URL | https://pubchem.ncbi.nlm.nih.gov | |
Description | Data deposited in or computed by PubChem | |
InChI Key |
YRYOXRMDHALAFL-QMMMGPOBSA-N | |
Source | PubChem | |
URL | https://pubchem.ncbi.nlm.nih.gov | |
Description | Data deposited in or computed by PubChem | |
Canonical SMILES |
CCCC(=O)CC(=O)NC1CCOC1=O | |
Source | PubChem | |
URL | https://pubchem.ncbi.nlm.nih.gov | |
Description | Data deposited in or computed by PubChem | |
Isomeric SMILES |
CCCC(=O)CC(=O)N[C@H]1CCOC1=O | |
Source | PubChem | |
URL | https://pubchem.ncbi.nlm.nih.gov | |
Description | Data deposited in or computed by PubChem | |
Molecular Formula |
C10H15NO4 | |
Source | PubChem | |
URL | https://pubchem.ncbi.nlm.nih.gov | |
Description | Data deposited in or computed by PubChem | |
Molecular Weight |
213.23 g/mol | |
Source | PubChem | |
URL | https://pubchem.ncbi.nlm.nih.gov | |
Description | Data deposited in or computed by PubChem | |
Retrosynthesis Analysis
AI-Powered Synthesis Planning: Our tool employs the Template_relevance Pistachio, Template_relevance Bkms_metabolic, Template_relevance Pistachio_ringbreaker, Template_relevance Reaxys, Template_relevance Reaxys_biocatalysis model, leveraging a vast database of chemical reactions to predict feasible synthetic routes.
One-Step Synthesis Focus: Specifically designed for one-step synthesis, it provides concise and direct routes for your target compounds, streamlining the synthesis process.
Accurate Predictions: Utilizing the extensive PISTACHIO, BKMS_METABOLIC, PISTACHIO_RINGBREAKER, REAXYS, REAXYS_BIOCATALYSIS database, our tool offers high-accuracy predictions, reflecting the latest in chemical research and data.
Strategy Settings
Precursor scoring | Relevance Heuristic |
---|---|
Min. plausibility | 0.01 |
Model | Template_relevance |
Template Set | Pistachio/Bkms_metabolic/Pistachio_ringbreaker/Reaxys/Reaxys_biocatalysis |
Top-N result to add to graph | 6 |
Feasible Synthetic Routes
Disclaimer and Information on In-Vitro Research Products
Please be aware that all articles and product information presented on BenchChem are intended solely for informational purposes. The products available for purchase on BenchChem are specifically designed for in-vitro studies, which are conducted outside of living organisms. In-vitro studies, derived from the Latin term "in glass," involve experiments performed in controlled laboratory settings using cells or tissues. It is important to note that these products are not categorized as medicines or drugs, and they have not received approval from the FDA for the prevention, treatment, or cure of any medical condition, ailment, or disease. We must emphasize that any form of bodily introduction of these products into humans or animals is strictly prohibited by law. It is essential to adhere to these guidelines to ensure compliance with legal and ethical standards in research and experimentation.