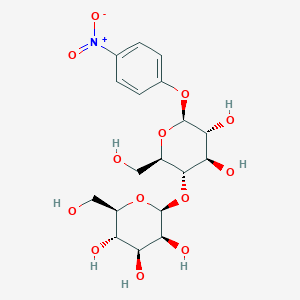
Man1-b-4-Glc-OPNP
- Click on QUICK INQUIRY to receive a quote from our team of experts.
- With the quality product at a COMPETITIVE price, you can focus more on your research.
Overview
Description
Man1-b-4-Glc-OPNP, also known as 4-Nitrophenyl β-D-mannopyranosyl-(1→4)-β-D-glucopyranoside, is a synthetic glycoside. This compound is notable for its role in biochemical research, particularly in the study of glycosidase enzymes. It consists of a mannose and glucose moiety linked through a β-1,4-glycosidic bond, with a 4-nitrophenyl group attached to the glucose unit.
Preparation Methods
Synthetic Routes and Reaction Conditions
The synthesis of Man1-b-4-Glc-OPNP typically involves the following steps:
Glycosylation Reaction: The initial step involves the glycosylation of a glucose derivative with a mannose donor. This reaction is often catalyzed by a Lewis acid such as boron trifluoride etherate (BF3·Et2O) under anhydrous conditions.
Introduction of the 4-Nitrophenyl Group: The 4-nitrophenyl group is introduced through a nucleophilic substitution reaction. This involves reacting the glycosylated product with 4-nitrophenol in the presence of a base like sodium hydride (NaH) or potassium carbonate (K2CO3).
Industrial Production Methods
Industrial production of this compound follows similar synthetic routes but on a larger scale. The process is optimized for higher yields and purity, often involving automated synthesis and purification techniques such as high-performance liquid chromatography (HPLC).
Chemical Reactions Analysis
Types of Reactions
Man1-b-4-Glc-OPNP undergoes several types of chemical reactions:
Hydrolysis: This compound is susceptible to hydrolysis, particularly under acidic or basic conditions, leading to the cleavage of the glycosidic bond.
Oxidation: The nitrophenyl group can undergo oxidation reactions, often resulting in the formation of nitro derivatives.
Reduction: The nitrophenyl group can also be reduced to an amino group using reducing agents like sodium borohydride (NaBH4).
Common Reagents and Conditions
Hydrolysis: Acidic conditions (e.g., hydrochloric acid) or enzymatic hydrolysis using glycosidases.
Oxidation: Oxidizing agents such as potassium permanganate (KMnO4) or hydrogen peroxide (H2O2).
Reduction: Reducing agents like sodium borohydride (NaBH4) or catalytic hydrogenation.
Major Products Formed
Hydrolysis: Mannose and glucose.
Oxidation: Nitro derivatives of the phenyl group.
Reduction: Aminophenyl derivatives.
Scientific Research Applications
Man1-b-4-Glc-OPNP is widely used in scientific research due to its unique properties:
Biochemistry: It serves as a substrate for studying glycosidase enzymes, which are crucial in carbohydrate metabolism.
Medicine: Research involving this compound helps in understanding enzyme deficiencies and developing enzyme replacement therapies.
Industrial Applications: It is used in the synthesis of complex carbohydrates and glycoconjugates, which have applications in pharmaceuticals and biotechnology.
Mechanism of Action
The mechanism of action of Man1-b-4-Glc-OPNP involves its interaction with glycosidase enzymes. The compound binds to the active site of the enzyme, where the glycosidic bond is cleaved, releasing the 4-nitrophenyl group. This reaction can be monitored spectrophotometrically, as the 4-nitrophenyl group absorbs light at a specific wavelength, allowing for the quantification of enzyme activity.
Comparison with Similar Compounds
Similar Compounds
4-Nitrophenyl β-D-glucopyranoside: Similar structure but lacks the mannose moiety.
4-Nitrophenyl β-D-galactopyranoside: Contains a galactose unit instead of mannose.
4-Nitrophenyl β-D-mannopyranoside: Lacks the glucose moiety.
Uniqueness
Man1-b-4-Glc-OPNP is unique due to its dual sugar moieties (mannose and glucose) linked by a β-1,4-glycosidic bond, making it a valuable tool for studying specific glycosidase activities that act on such linkages. Its 4-nitrophenyl group also provides a convenient spectrophotometric handle for enzyme assays.
This comprehensive overview should provide a solid foundation for understanding this compound, its synthesis, reactions, applications, and how it compares to similar compounds
Properties
Molecular Formula |
C18H25NO13 |
---|---|
Molecular Weight |
463.4 g/mol |
IUPAC Name |
(2S,3S,4S,5S,6R)-2-[(2R,3S,4R,5R,6S)-4,5-dihydroxy-2-(hydroxymethyl)-6-(4-nitrophenoxy)oxan-3-yl]oxy-6-(hydroxymethyl)oxane-3,4,5-triol |
InChI |
InChI=1S/C18H25NO13/c20-5-9-11(22)12(23)14(25)18(30-9)32-16-10(6-21)31-17(15(26)13(16)24)29-8-3-1-7(2-4-8)19(27)28/h1-4,9-18,20-26H,5-6H2/t9-,10-,11-,12+,13-,14+,15-,16-,17-,18+/m1/s1 |
InChI Key |
IAYJZWFYUSNIPN-VKDBYXODSA-N |
Isomeric SMILES |
C1=CC(=CC=C1[N+](=O)[O-])O[C@H]2[C@@H]([C@H]([C@@H]([C@H](O2)CO)O[C@H]3[C@H]([C@H]([C@@H]([C@H](O3)CO)O)O)O)O)O |
Canonical SMILES |
C1=CC(=CC=C1[N+](=O)[O-])OC2C(C(C(C(O2)CO)OC3C(C(C(C(O3)CO)O)O)O)O)O |
Origin of Product |
United States |
Disclaimer and Information on In-Vitro Research Products
Please be aware that all articles and product information presented on BenchChem are intended solely for informational purposes. The products available for purchase on BenchChem are specifically designed for in-vitro studies, which are conducted outside of living organisms. In-vitro studies, derived from the Latin term "in glass," involve experiments performed in controlled laboratory settings using cells or tissues. It is important to note that these products are not categorized as medicines or drugs, and they have not received approval from the FDA for the prevention, treatment, or cure of any medical condition, ailment, or disease. We must emphasize that any form of bodily introduction of these products into humans or animals is strictly prohibited by law. It is essential to adhere to these guidelines to ensure compliance with legal and ethical standards in research and experimentation.