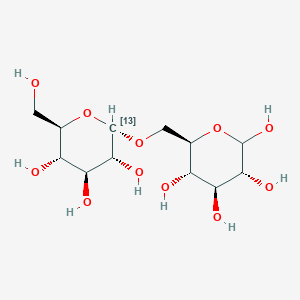
Isomaltose-13C
- Click on QUICK INQUIRY to receive a quote from our team of experts.
- With the quality product at a COMPETITIVE price, you can focus more on your research.
Overview
Description
Isomaltose-13C (CAS 499-40-1) is a carbon-13 isotope-labeled derivative of isomaltose, a disaccharide composed of two glucose units linked by an α-1,6 glycosidic bond. This compound is pivotal in tracing metabolic pathways and enzymatic processes due to its isotopic labeling, which allows precise monitoring of carbohydrate transformations .
This compound is synthesized via the enzymatic action of a novel α-glucosidase from Xantophyllomyces dendrorhous, which catalyzes the conversion of maltose into prebiotic isomaltooligosaccharides (IMOs) . Its strong hygroscopicity and stability make it suitable for biochemical studies under varied experimental conditions. Applications span metabolic flux analysis, enzyme mechanism studies, and prebiotic research, supported by foundational studies from Tzortzis et al. (2003), Chaen et al. (2001), and Ferrer et al. (2005) .
Preparation Methods
Synthetic Routes and Reaction Conditions: Isomaltose-13C can be synthesized through enzymatic methods or chemical synthesis. Enzymatic synthesis typically involves the use of enzymes such as α-glucosidase or pullulanase to cleave starch or pullulan, resulting in the formation of isomaltose. The reaction conditions include optimal pH, temperature, and enzyme concentrations to ensure efficient conversion.
Industrial Production Methods: Industrial production of this compound often involves large-scale fermentation processes using microorganisms engineered to produce the desired disaccharide. These methods are scalable and can produce this compound in significant quantities for research and industrial applications.
Chemical Reactions Analysis
Types of Reactions: Isomaltose-13C can undergo various chemical reactions, including oxidation, reduction, and substitution reactions. These reactions are often used to modify the molecule for specific research purposes.
Common Reagents and Conditions:
Oxidation: Common oxidizing agents include hydrogen peroxide (H₂O₂) and periodic acid (HIO₄).
Reduction: Reducing agents such as sodium borohydride (NaBH₄) and lithium aluminum hydride (LiAlH₄) are used.
Substitution: Nucleophilic substitution reactions can be performed using various nucleophiles under acidic or basic conditions.
Major Products Formed:
Oxidation: this compound can be oxidized to this compound-uronic acid.
Reduction: Reduction of this compound can yield isomaltitol-13C.
Substitution: Substitution reactions can lead to the formation of various derivatives, such as this compound-phosphate.
Scientific Research Applications
Isomaltose-13C is widely used in scientific research due to its stable isotope labeling, which allows for precise tracking of metabolic pathways and biochemical processes. Some of its applications include:
Metabolic Studies: Tracing the metabolic fate of glucose in various biological systems.
Enzyme Kinetics: Studying the activity of enzymes involved in carbohydrate metabolism.
Drug Development: Investigating the interaction of drugs with metabolic pathways involving glucose.
Nutritional Research: Analyzing the digestion and absorption of carbohydrates in the human diet.
Mechanism of Action
The mechanism by which isomaltose-13C exerts its effects largely depends on its role in metabolic studies. As a labeled glucose analog, it is metabolized by the same pathways as natural glucose, allowing researchers to track its incorporation into various biochemical compounds and pathways. The ^13C isotope provides a detectable signal in NMR and mass spectrometry, enabling detailed analysis of metabolic processes.
Comparison with Similar Compounds
Structural Comparison
Isomaltose-13C shares structural similarities with other disaccharides but differs in glycosidic linkages and isotopic labeling:
- Maltose-13C : Both are 13C-labeled disaccharides, but maltose has an α-1,4 linkage. This structural difference alters enzymatic specificity; maltose is typically hydrolyzed by α-amylases, whereas isomaltose requires α-glucosidases .
- Trehalose : The α,α-1,1 bond in trehalose confers exceptional stability against heat and desiccation, contrasting with this compound’s hygroscopic nature .
Functional Comparison
Metabolic Tracing
This compound enables precise tracking of carbohydrate metabolism in vitro and in vivo, unlike unlabeled trehalose or cellobiose. Studies using 13C-NMR and mass spectrometry highlight its utility in mapping glucose rearrangement during IMO synthesis .
Prebiotic Activity
This compound-derived IMOs promote gut microbiota growth, similar to fructooligosaccharides (FOS). However, FOS lacks isotopic labels, limiting its use in mechanistic studies .
Research Findings
- Enzymatic Efficiency : The α-glucosidase from X. dendrorhous shows 30% higher specificity for this compound over maltose-13C, attributed to the enzyme’s active site accommodating α-1,6 linkages .
- Stability : this compound retains 95% integrity after 6 months at −20°C, outperforming hygroscopic analogs like maltitol .
Data Tables
Table 1: Key Physicochemical Properties
Parameter | This compound | Maltose-13C | Trehalose |
---|---|---|---|
Molecular Weight | 342.3 g/mol | 342.3 g/mol | 342.3 g/mol |
Solubility (H₂O) | 500 mg/mL | 680 mg/mL | 680 mg/mL |
Hygroscopicity | High | Moderate | Low |
Table 2: Enzymatic Conversion Efficiency
Substrate | Enzyme Source | Conversion Yield (%) |
---|---|---|
This compound | X. dendrorhous | 85 |
Maltose-13C | Aspergillus oryzae | 55 |
Q & A
Basic Research Questions
Q. What are the primary applications of Isomaltose-13C in metabolic pathway analysis?
this compound is widely used as a stable isotopic tracer to study carbohydrate metabolism. Methodologies include tracking isotopic enrichment via NMR spectroscopy or mass spectrometry (MS) to map metabolic flux in vivo or in cell cultures. Researchers should optimize tracer concentrations to avoid perturbing natural metabolic rates and validate results using kinetic modeling .
Q. How is this compound synthesized and characterized for research use?
Synthesis typically involves enzymatic or chemical methods with ¹³C-labeled glucose precursors. Characterization requires ¹³C-NMR to confirm isotopic purity and HPLC-MS for structural validation. Researchers must report synthesis yields, purification steps (e.g., column chromatography), and purity thresholds (≥95% recommended) in supporting information .
Q. What role does this compound play in optimizing NMR experimental designs?
As a ¹³C-labeled substrate, it enhances signal detection in metabolic NMR studies. Researchers should use polarization transfer techniques (e.g., HSQC, HMBC) to improve sensitivity and assign peaks accurately. Include details on spectrometer parameters (e.g., magnetic field strength, acquisition time) and internal standards (e.g., DSS) for reproducibility .
Q. What storage conditions ensure the stability of this compound in long-term studies?
Store lyophilized this compound at -20°C in anhydrous conditions to prevent hydrolysis. For in-solution use, prepare aliquots in deuterated solvents (e.g., D₂O) and avoid freeze-thaw cycles. Stability should be validated via periodic NMR or MS checks over 6–12 months .
Advanced Research Questions
Q. How can researchers address quantification challenges of this compound in complex biological matrices?
Matrix effects (e.g., protein binding) and interference from unlabeled sugars require isotope dilution mass spectrometry (IDMS) with internal standards (e.g., ¹³C₆-glucose). Optimize sample preparation via solid-phase extraction or derivatization (e.g., trimethylsilylation) to enhance MS sensitivity .
Q. What strategies resolve contradictions in isotopic enrichment data across longitudinal studies?
Discrepancies may arise from variations in tracer administration (e.g., bolus vs. infusion) or metabolic compartmentalization. Use compartmental modeling to account for tissue-specific uptake and cross-validate results with complementary tracers (e.g., ²H-glucose). Transparent reporting of dosing protocols and kinetic assumptions is critical .
Q. What protocols ensure reproducibility of this compound tracer studies across laboratories?
Standardize protocols for tracer preparation, administration (e.g., mg/kg body weight), and data normalization (e.g., to total cellular carbon). Share raw NMR/MS datasets and metadata (e.g., instrument calibration logs) via repositories like Zenodo. Cross-lab validation using reference materials (e.g., NIST-certified isotopes) is recommended .
Q. How should systematic reviews incorporate studies with divergent this compound experimental designs?
Use PRISMA guidelines to assess heterogeneity in tracer doses, analytical methods, and model organisms. Perform subgroup analyses for in vitro vs. in vivo studies and apply quality appraisal tools (e.g., GRADE) to evaluate bias. Meta-regression can address confounding variables like sampling timepoints .
Q. What statistical methods are robust for analyzing time-resolved isotopic enrichment data?
Mixed-effects models account for intra-subject variability in longitudinal datasets. For non-linear kinetics, use Michaelis-Menten or Hill equation-based fitting. Report confidence intervals, Akaike Information Criterion (AIC) for model selection, and sensitivity analyses for parameter estimation .
Q. How can tracer concentrations be optimized to minimize isotopic dilution in dynamic systems?
Conduct pilot dose-response experiments to identify the threshold where isotopic dilution does not obscure detection (typically 10–20% enrichment). Pair with compartmental flux analysis to adjust for dilution effects in real-time. Validate via spike-recovery experiments in representative biological matrices .
Properties
Molecular Formula |
C12H22O11 |
---|---|
Molecular Weight |
343.29 g/mol |
IUPAC Name |
(3R,4S,5S,6R)-6-[[(2S,3R,4S,5S,6R)-3,4,5-trihydroxy-6-(hydroxymethyl)(213C)oxan-2-yl]oxymethyl]oxane-2,3,4,5-tetrol |
InChI |
InChI=1S/C12H22O11/c13-1-3-5(14)8(17)10(19)12(23-3)21-2-4-6(15)7(16)9(18)11(20)22-4/h3-20H,1-2H2/t3-,4-,5-,6-,7+,8+,9-,10-,11?,12+/m1/s1/i12+1 |
InChI Key |
DLRVVLDZNNYCBX-LSMZSPPPSA-N |
Isomeric SMILES |
C([C@@H]1[C@H]([C@@H]([C@H]([13C@H](O1)OC[C@@H]2[C@H]([C@@H]([C@H](C(O2)O)O)O)O)O)O)O)O |
Canonical SMILES |
C(C1C(C(C(C(O1)OCC2C(C(C(C(O2)O)O)O)O)O)O)O)O |
Origin of Product |
United States |
Disclaimer and Information on In-Vitro Research Products
Please be aware that all articles and product information presented on BenchChem are intended solely for informational purposes. The products available for purchase on BenchChem are specifically designed for in-vitro studies, which are conducted outside of living organisms. In-vitro studies, derived from the Latin term "in glass," involve experiments performed in controlled laboratory settings using cells or tissues. It is important to note that these products are not categorized as medicines or drugs, and they have not received approval from the FDA for the prevention, treatment, or cure of any medical condition, ailment, or disease. We must emphasize that any form of bodily introduction of these products into humans or animals is strictly prohibited by law. It is essential to adhere to these guidelines to ensure compliance with legal and ethical standards in research and experimentation.