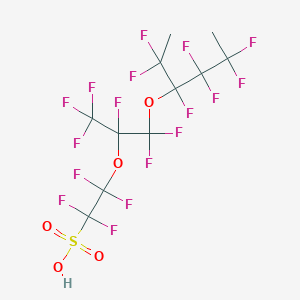
Nafion (Technical Grade)
- Click on QUICK INQUIRY to receive a quote from our team of experts.
- With the quality product at a COMPETITIVE price, you can focus more on your research.
Overview
Description
Nafion (Technical Grade) is a perfluorinated sulfonic acid (PFSA) ion-exchange resin with the CAS number 31175-20-9 . It is widely used in electrochemical applications, including fuel cells, catalytic membranes, and sensor technologies, due to its exceptional proton conductivity, chemical stability, and mechanical durability . Technical Grade Nafion is produced under standardized protocols to ensure consistency for industrial applications, balancing cost-effectiveness with performance . Unlike higher-purity grades (e.g., ACS or Reagent Grade), Technical Grade prioritizes functional performance over analytical precision, making it suitable for large-scale processes like catalyst ink preparation .
Preparation Methods
Synthetic Routes and Reaction Conditions: Nafion is synthesized through the copolymerization of tetrafluoroethylene (TFE) and a perfluoro (alkyl vinyl ether) sulfonyl fluoride monomer. The reaction typically occurs under free-radical polymerization conditions, often initiated by agents like persulfate or azo compounds.
Industrial Production Methods: Industrial production of Nafion involves scaling up the polymerization process, ensuring consistent quality and performance. The resulting polymer is then processed into membranes or other forms suitable for various applications.
Chemical Reactions Analysis
Types of Reactions: Nafion undergoes several types of reactions, including:
Oxidation: Nafion can be oxidized to form sulfonic acid groups, enhancing its ion-exchange capacity.
Reduction: Reduction reactions can modify the polymer's properties, such as improving its mechanical strength.
Substitution: Substitution reactions can introduce different functional groups, altering its chemical behavior.
Common Reagents and Conditions:
Oxidation: Common oxidizing agents include hydrogen peroxide and potassium permanganate.
Reduction: Reducing agents like sodium borohydride are often used.
Substitution: Various reagents, depending on the desired functional group, are employed under controlled conditions.
Major Products Formed:
Oxidation: Sulfonic acid derivatives.
Reduction: Reduced forms of Nafion with modified properties.
Substitution: Functionalized Nafion derivatives with tailored chemical properties.
Scientific Research Applications
Nafion (Technical Grade) is a perfluorosulfonic acid (PFSA) membrane that has various applications in chemical technologies . It is a widely used material for polyelectrolyte membranes and serves as a standard for comparison against novel materials .
General Properties and Characteristics:
- Composition: Nafion is composed of a perfluorinated matrix and side chains, giving it unique thermal and chemical properties .
- Thermal Stability: Thermogravimetric analysis reveals three stages of thermal degradation in Nafion, corresponding to water loss, C-S bond cleavage, and perfluorinated matrix decomposition .
- Ionic Properties: Sulfonic groups in Nafion are responsible for its luminescence properties .
Applications:
- PEM Fuel Cells: Nafion is used in proton exchange membrane (PEM) fuel cells, where it functions within a wide temperature range . It is used in fuel cells designed to operate above 100°C to enhance electrochemical kinetics, simplify cooling systems, facilitate water management, and improve system CO tolerance . However, high temperatures can accelerate membrane degradation, raising concerns about the thermal stability of the Nafion electrolyte .
- Electrolysis: Nafion is used as a membrane in various electrolysis processes .
- Chemical Technologies: Due to its properties, Nafion is utilized in various chemical technologies .
- Research and Development: Nafion is used in research, production, testing laboratories, healthcare, and science education .
- Other potential applications: biological applications .
Environmental Concerns:
- PFC Production: The thermal decomposition of Nafion can produce perfluorinated compounds (PFCs), including perfluorocarboxylic acids, which can contribute to environmental pollution .
- Waste Disposal: Incineration of Nafion components may produce undesirable thermal decomposition products, including PFCs, which are a long-term environmental challenge .
Analytical Techniques:
- LC/ESI-MS/MS: Liquid chromatography-electrospray ionization-tandem mass spectrometry is used for structural identification of thermolysis products .
- Ion Chromatography: Used to study fluoride release during thermolysis .
- Thermogravimetric Analysis (TGA): Used to characterize the membrane's thermal stability .
- FTIR Spectroscopy: Used to study the spectral range of λ = 1.8–2.2 μm.
Factors Affecting Performance and Durability:
- High Temperatures: Operation at high temperatures can accelerate membrane degradation .
- Water Management: Water content affects Nafion swelling .
- Radical Attack: Radical species can trigger the decomposition of PEMs under realistic operating conditions .
- Electron Beam Irradiation: Changes the elemental composition of Nafion film .
Mechanism of Action
Nafion exerts its effects primarily through its ion-exchange properties. The sulfonic acid groups within the polymer matrix facilitate the transport of cations, making it an effective proton exchange membrane. The molecular pathways involved include the movement of protons through the hydrated polymer network, which is crucial for its function in fuel cells and other electrochemical applications.
Comparison with Similar Compounds
Nafion (Technical Grade) vs. Other Nafion Grades
Nafion is available in multiple grades tailored to specific applications:
Technical Grade is preferred for industrial applications requiring bulk material, while ACS/Reagent Grade is used for research requiring stringent purity .
Nafion vs. Nafion-Based Composites
Nafion is often modified with nanomaterials to enhance performance. Key comparisons from high-temperature hydrogen-air fuel cell (HT-HAFC) studies include:
Key Findings :
- The addition of sulfonated unzipped graphite nanofibers (SO3H-UGNF) improves proton conductivity and reduces hydrogen crossover due to enhanced interfacial interactions .
- Bare Nafion exhibits higher hydrogen permeability, limiting its efficiency in fuel cells compared to composites .
Research Insights and Limitations
- Mechanical Properties : Nafion’s hydration-dependent behavior, modeled as a composite of crystalline, amorphous, and water phases, distinguishes it from rigid polymers like polyethylene .
- Industrial vs. Research Use : Technical Grade’s lower purity may introduce trace impurities affecting reproducibility in academic studies, necessitating higher grades for controlled experiments .
- Data Gaps: Direct comparisons with non-Nafion PFSA membranes (e.g., Aquivion®) are absent in the provided evidence, highlighting a need for expanded studies .
Biological Activity
Nafion, a perfluorinated sulfonic acid ionomer, is widely recognized for its applications in fuel cells and other electrochemical devices due to its unique properties, including high ionic conductivity, chemical stability, and mechanical strength. However, its biological activity has garnered attention in recent years, particularly concerning its biocompatibility and potential toxicity. This article reviews the biological activity of Nafion (Technical Grade), focusing on its interactions with biological systems, relevant case studies, and research findings.
Structure and Properties of Nafion
Nafion consists of a hydrophobic tetrafluoroethylene backbone with hydrophilic sulfonic acid groups that facilitate proton conduction. The unique structure allows for the formation of water-filled channels that enhance ionic transport. The chemical formula can be represented as:
This dissociation is crucial for understanding its role in various applications, including fuel cells and as a potential biomaterial .
Biocompatibility Studies
Recent studies have evaluated the biocompatibility of Nafion and its composites. For instance, a study on PEDOT:Nafion coatings demonstrated promising results regarding cell adhesion and proliferation. Rat fibroblasts cultured on these coatings showed good viability over a period of 7 days, indicating that Nafion-based materials may support cellular functions effectively .
Table 1: Summary of Biocompatibility Findings
Study | Material | Cell Type | Viability (%) | Duration (Days) |
---|---|---|---|---|
PEDOT:Nafion | Rat fibroblasts | 85-90 | 7 | |
Nafion/SiO2 | Human endothelial cells | >80 | 5 |
Toxicological Concerns
While Nafion shows potential as a biocompatible material, concerns have been raised regarding its byproducts. Nafion byproduct 2 (NBP2) has been identified as a contaminant in various environmental samples. Research indicates that NBP2 exposure in pregnant rats led to developmental toxicity, including stillbirths and reduced pup survival rates . This emphasizes the need for careful consideration of Nafion's environmental impact and the safety of its byproducts in biological contexts.
Case Studies and Research Findings
Several studies have explored the biological interactions of Nafion:
- In Vitro Cytotoxicity : A study assessing the cytotoxicity of PEDOT:Nafion coatings found that while initial cell viability was high, long-term effects remain to be fully elucidated. This underscores the importance of extended studies to evaluate chronic exposure impacts .
- Water Uptake and States : Research utilizing terahertz spectroscopy revealed insights into water uptake in Nafion membranes, which is critical for understanding how hydration affects biological interactions . The ability of Nafion to retain water is essential for maintaining its ionic conductivity and could influence its interaction with biological tissues.
- Modification Effects : Investigations into Nafion/SiO2 composites demonstrated improved physical properties and water retention capabilities, which could enhance their applicability in biomedical devices while potentially mitigating toxicity risks associated with pure Nafion .
Q & A
Basic Research Questions
Q. What are the standard methodologies for characterizing the physicochemical properties of Nafion (Technical Grade)?
- Methodological Answer : Key techniques include Fourier-transform infrared spectroscopy (FTIR) to analyze sulfonic acid group distribution, scanning electron microscopy (SEM) for membrane morphology, and electrochemical impedance spectroscopy (EIS) for proton conductivity measurements. Hydration-dependent conductivity experiments should be conducted at controlled humidity levels (e.g., 20–90% RH) and temperatures (25–80°C) to replicate operational conditions . For thermal stability, thermogravimetric analysis (TGA) under inert atmospheres is recommended.
Q. How should researchers design experiments to assess Nafion’s ion-exchange capacity (IEC) and hydration behavior?
- Methodological Answer : IEC can be determined via titration using NaOH to neutralize sulfonic acid groups, followed by back-titration with HCl. Hydration studies require gravimetric analysis under controlled humidity chambers, with data normalized to dry membrane mass. Ensure variables like pretreatment (e.g., boiling in H₂O₂/H₂SO₄) are standardized to minimize batch-to-batch variability .
Q. What are best practices for integrating Nafion into electrochemical cells for fuel-cell testing?
- Methodological Answer : Use hot-pressing to laminate Nafion membranes between gas diffusion electrodes (GDEs) at 130–150°C under 50–100 psi pressure. Validate membrane-electrode assembly (MEA) integrity via cyclic voltammetry and polarization curves. Include control experiments with commercial Nafion membranes (e.g., Nafion 117) for benchmarking .
Advanced Research Questions
Q. How can researchers reconcile contradictory data on Nafion’s proton conductivity under low-humidity conditions?
- Methodological Answer : Contradictions often arise from variations in membrane pretreatment, measurement techniques (e.g., in-plane vs. through-plane conductivity), or water content calibration. Use deuterium NMR to probe water dynamics and compare with molecular dynamics (MD) simulations to validate mechanistic hypotheses. Replicate studies across multiple labs with shared protocols to isolate experimental artifacts .
Q. What advanced computational models are suitable for predicting Nafion’s nanostructure-property relationships?
- Methodological Answer : Coarse-grained MD simulations or density functional theory (DFT) can model sulfonic acid group clustering and water-channel formation. Pair simulations with small-angle X-ray scattering (SAXS) data to refine force fields. For phenomenological transport modeling, use Poisson-Boltzmann theory to link ionic conductivity to membrane hydration .
Q. How can researchers optimize Nafion-based composites for high-temperature PEM fuel cells?
- Methodological Answer : Incorporate inorganic fillers (e.g., SiO₂, TiO₂) via sol-gel methods to enhance thermal stability. Characterize composite homogeneity using energy-dispersive X-ray spectroscopy (EDS) and assess mechanical durability via dynamic mechanical analysis (DMA). Validate performance via accelerated stress tests (ASTs) under cyclic humidity/temperature conditions .
Q. What strategies address parasitic gas crossover in Nafion membranes during electrolyzer operation?
- Methodological Answer : Quantify crossover rates using hydrogen pump experiments or gas chromatography. Modify membranes with barrier layers (e.g., graphene oxide) or crosslink sulfonic acid groups to reduce porosity. Compare results with theoretical models (e.g., Fick’s law) to identify dominant transport mechanisms .
Q. Methodological and Analytical Frameworks
Q. How should researchers design a systematic review to evaluate Nafion’s degradation mechanisms in fuel cells?
- Methodological Answer : Follow PRISMA guidelines to identify peer-reviewed studies on chemical (radical attack) and mechanical (pinhole formation) degradation. Use meta-analysis tools to quantify correlations between operating conditions (e.g., voltage cycling) and lifetime. Apply GRADE criteria to assess evidence quality and identify gaps for future studies .
Q. What statistical approaches are recommended for analyzing variability in Nafion membrane batch performance?
- Methodological Answer : Use ANOVA to compare batch means for critical parameters (e.g., IEC, conductivity). Apply principal component analysis (PCA) to identify hidden variables (e.g., solvent purity, curing time) influencing performance. Report confidence intervals and effect sizes to contextualize practical significance .
Q. How can in-situ spectroscopic techniques enhance understanding of Nafion’s interfacial behavior in fuel cells?
- Methodological Answer : Use operando Raman spectroscopy to monitor sulfonic group degradation during voltage cycling. Pair with electrochemical atomic force microscopy (EC-AFM) to correlate chemical changes with morphological evolution. Data should be time-synchronized with cell voltage/current outputs to establish causality .
Q. Ethical and Reproducibility Considerations
Q. What protocols ensure reproducibility in Nafion membrane fabrication studies?
- Methodological Answer : Document all synthesis parameters (e.g., solvent type, drying temperature) using standardized templates (e.g., Materials Methods sections in ACS journals). Share raw data (e.g., TGA curves, EIS spectra) in public repositories like Zenodo. Collaborate with third-party labs for blind validation .
Q. How can researchers mitigate bias when reporting Nafion’s performance enhancements in composites?
Properties
Molecular Formula |
C11H7F17O5S |
---|---|
Molecular Weight |
574.21 g/mol |
IUPAC Name |
1,1,2,2-tetrafluoro-2-[1,1,1,2,3,3-hexafluoro-3-(2,2,3,4,4,5,5-heptafluorohexan-3-yloxy)propan-2-yl]oxyethanesulfonic acid |
InChI |
InChI=1S/C11H7F17O5S/c1-3(12,13)5(16,17)6(18,4(2,14)15)32-9(23,24)7(19,8(20,21)22)33-10(25,26)11(27,28)34(29,30)31/h1-2H3,(H,29,30,31) |
InChI Key |
WMZXFJWZVIJRFN-UHFFFAOYSA-N |
Canonical SMILES |
CC(C(C(C(C)(F)F)(F)F)(OC(C(C(F)(F)F)(OC(C(F)(F)S(=O)(=O)O)(F)F)F)(F)F)F)(F)F |
Origin of Product |
United States |
Disclaimer and Information on In-Vitro Research Products
Please be aware that all articles and product information presented on BenchChem are intended solely for informational purposes. The products available for purchase on BenchChem are specifically designed for in-vitro studies, which are conducted outside of living organisms. In-vitro studies, derived from the Latin term "in glass," involve experiments performed in controlled laboratory settings using cells or tissues. It is important to note that these products are not categorized as medicines or drugs, and they have not received approval from the FDA for the prevention, treatment, or cure of any medical condition, ailment, or disease. We must emphasize that any form of bodily introduction of these products into humans or animals is strictly prohibited by law. It is essential to adhere to these guidelines to ensure compliance with legal and ethical standards in research and experimentation.