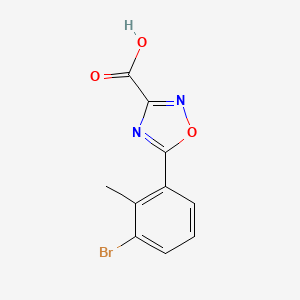
5-(3-Bromo-2-methylphenyl)-1,2,4-oxadiazole-3-carboxylic acid
- Click on QUICK INQUIRY to receive a quote from our team of experts.
- With the quality product at a COMPETITIVE price, you can focus more on your research.
Overview
Description
5-(3-Bromo-2-methylphenyl)-1,2,4-oxadiazole-3-carboxylic acid is a heterocyclic compound that features a 1,2,4-oxadiazole ring substituted with a 3-bromo-2-methylphenyl group and a carboxylic acid group
Preparation Methods
Synthetic Routes and Reaction Conditions
The synthesis of 5-(3-Bromo-2-methylphenyl)-1,2,4-oxadiazole-3-carboxylic acid typically involves the cyclization of appropriate precursors One common method involves the reaction of 3-bromo-2-methylbenzonitrile with hydroxylamine to form the corresponding amidoxime, which is then cyclized to form the oxadiazole ring
Industrial Production Methods
Industrial production methods for this compound may involve similar synthetic routes but are optimized for large-scale production. This includes the use of continuous flow reactors and automated systems to ensure consistent quality and yield.
Chemical Reactions Analysis
Types of Reactions
5-(3-Bromo-2-methylphenyl)-1,2,4-oxadiazole-3-carboxylic acid undergoes various types of chemical reactions, including:
Substitution Reactions: The bromine atom can be substituted with other nucleophiles.
Oxidation and Reduction Reactions: The oxadiazole ring can participate in redox reactions under specific conditions.
Hydrolysis: The carboxylic acid group can be esterified or converted to other derivatives.
Common Reagents and Conditions
Substitution Reactions: Common reagents include nucleophiles such as amines or thiols, often under basic conditions.
Oxidation and Reduction Reactions: Reagents such as hydrogen peroxide or sodium borohydride can be used.
Hydrolysis: Acidic or basic conditions can be employed to hydrolyze ester derivatives to the carboxylic acid.
Major Products
Substitution Reactions: Products include derivatives where the bromine atom is replaced by other functional groups.
Oxidation and Reduction Reactions: Products include oxidized or reduced forms of the oxadiazole ring.
Hydrolysis: Products include the carboxylic acid or its derivatives.
Scientific Research Applications
5-(3-Bromo-2-methylphenyl)-1,2,4-oxadiazole-3-carboxylic acid has several scientific research applications:
Medicinal Chemistry: It is investigated for its potential as a pharmacophore in drug design, particularly for its antimicrobial and anticancer properties.
Materials Science: The compound is studied for its potential use in organic electronics and as a building block for advanced materials.
Biological Research: It is used as a probe to study biological pathways and interactions due to its unique chemical properties.
Mechanism of Action
The mechanism of action of 5-(3-Bromo-2-methylphenyl)-1,2,4-oxadiazole-3-carboxylic acid involves its interaction with specific molecular targets. In medicinal chemistry, it may inhibit enzymes or receptors involved in disease pathways. The oxadiazole ring can interact with biological macromolecules through hydrogen bonding and hydrophobic interactions, influencing the compound’s biological activity.
Comparison with Similar Compounds
Similar Compounds
- 3-Bromo-2-methylbenzoic acid
- 3-Bromo-2-methylphenylboronic acid
- 5-Bromo-2-methyl-1,3,4-oxadiazole
Uniqueness
5-(3-Bromo-2-methylphenyl)-1,2,4-oxadiazole-3-carboxylic acid is unique due to the presence of both the oxadiazole ring and the carboxylic acid group, which confer distinct chemical and biological properties. Its structure allows for versatile chemical modifications, making it a valuable compound in various research fields.
Properties
Molecular Formula |
C10H7BrN2O3 |
---|---|
Molecular Weight |
283.08 g/mol |
IUPAC Name |
5-(3-bromo-2-methylphenyl)-1,2,4-oxadiazole-3-carboxylic acid |
InChI |
InChI=1S/C10H7BrN2O3/c1-5-6(3-2-4-7(5)11)9-12-8(10(14)15)13-16-9/h2-4H,1H3,(H,14,15) |
InChI Key |
HVQKGASWQKZNKB-UHFFFAOYSA-N |
Canonical SMILES |
CC1=C(C=CC=C1Br)C2=NC(=NO2)C(=O)O |
Origin of Product |
United States |
Disclaimer and Information on In-Vitro Research Products
Please be aware that all articles and product information presented on BenchChem are intended solely for informational purposes. The products available for purchase on BenchChem are specifically designed for in-vitro studies, which are conducted outside of living organisms. In-vitro studies, derived from the Latin term "in glass," involve experiments performed in controlled laboratory settings using cells or tissues. It is important to note that these products are not categorized as medicines or drugs, and they have not received approval from the FDA for the prevention, treatment, or cure of any medical condition, ailment, or disease. We must emphasize that any form of bodily introduction of these products into humans or animals is strictly prohibited by law. It is essential to adhere to these guidelines to ensure compliance with legal and ethical standards in research and experimentation.