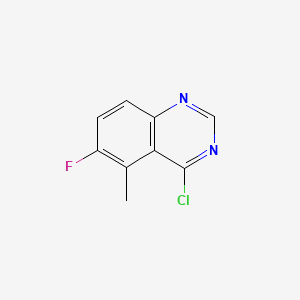
4-Chloro-6-fluoro-5-methylquinazoline
- Click on QUICK INQUIRY to receive a quote from our team of experts.
- With the quality product at a COMPETITIVE price, you can focus more on your research.
Overview
Description
4-Chloro-6-fluoro-5-methylquinazoline is a heterocyclic compound that belongs to the quinazoline family. Quinazolines are known for their diverse biological activities and are widely used in medicinal chemistry. The presence of chlorine, fluorine, and methyl groups in the quinazoline ring enhances its chemical properties, making it a valuable compound for various applications.
Preparation Methods
Synthetic Routes and Reaction Conditions: The synthesis of 4-chloro-6-fluoro-5-methylquinazoline typically involves the cyclization of appropriate precursors under specific conditions. One common method is the reaction of 4-chloro-2-fluoroaniline with methyl anthranilate in the presence of a dehydrating agent like phosphorus oxychloride (POCl3). The reaction is carried out under reflux conditions to facilitate the formation of the quinazoline ring.
Industrial Production Methods: Industrial production of this compound may involve similar synthetic routes but on a larger scale. The use of continuous flow reactors and optimized reaction conditions can enhance the yield and purity of the compound. Additionally, the use of green chemistry principles, such as solvent-free reactions and recyclable catalysts, can make the process more sustainable.
Chemical Reactions Analysis
Types of Reactions: 4-Chloro-6-fluoro-5-methylquinazoline undergoes various chemical reactions, including:
Substitution Reactions: The chlorine and fluorine atoms can be substituted with other functional groups using nucleophilic substitution reactions.
Oxidation and Reduction Reactions: The compound can undergo oxidation to form quinazoline N-oxides or reduction to form dihydroquinazolines.
Coupling Reactions: It can participate in Suzuki-Miyaura coupling reactions to form biaryl derivatives.
Common Reagents and Conditions:
Nucleophilic Substitution: Reagents like sodium methoxide or potassium tert-butoxide in polar aprotic solvents.
Oxidation: Reagents like hydrogen peroxide or m-chloroperbenzoic acid (m-CPBA).
Reduction: Reagents like sodium borohydride or lithium aluminum hydride.
Coupling: Palladium catalysts and boronic acids under mild conditions.
Major Products:
- Substituted quinazolines
- Quinazoline N-oxides
- Dihydroquinazolines
- Biaryl derivatives
Scientific Research Applications
4-Chloro-6-fluoro-5-methylquinazoline has a wide range of applications in scientific research:
Chemistry: Used as a building block for the synthesis of more complex molecules.
Biology: Studied for its potential as an enzyme inhibitor and its interactions with biological macromolecules.
Medicine: Investigated for its anticancer, antimicrobial, and anti-inflammatory properties.
Industry: Used in the development of agrochemicals and pharmaceuticals.
Mechanism of Action
The mechanism of action of 4-chloro-6-fluoro-5-methylquinazoline involves its interaction with specific molecular targets. For instance, it can inhibit tyrosine kinases by binding to the ATP-binding site, thereby blocking the phosphorylation of target proteins. This inhibition can lead to the suppression of cancer cell proliferation and survival. The presence of chlorine and fluorine atoms enhances its binding affinity and selectivity towards the target enzymes.
Comparison with Similar Compounds
4-Chloro-6-fluoro-5-methylquinazoline can be compared with other quinazoline derivatives such as:
- 4-Chloroquinazoline
- 6-Fluoroquinazoline
- 5-Methylquinazoline
Uniqueness: The combination of chlorine, fluorine, and methyl groups in this compound provides unique chemical properties, such as increased lipophilicity and enhanced binding affinity to molecular targets. This makes it a valuable compound for drug development and other applications.
Properties
Molecular Formula |
C9H6ClFN2 |
---|---|
Molecular Weight |
196.61 g/mol |
IUPAC Name |
4-chloro-6-fluoro-5-methylquinazoline |
InChI |
InChI=1S/C9H6ClFN2/c1-5-6(11)2-3-7-8(5)9(10)13-4-12-7/h2-4H,1H3 |
InChI Key |
BFXXRFICSKNRHT-UHFFFAOYSA-N |
Canonical SMILES |
CC1=C(C=CC2=C1C(=NC=N2)Cl)F |
Origin of Product |
United States |
Disclaimer and Information on In-Vitro Research Products
Please be aware that all articles and product information presented on BenchChem are intended solely for informational purposes. The products available for purchase on BenchChem are specifically designed for in-vitro studies, which are conducted outside of living organisms. In-vitro studies, derived from the Latin term "in glass," involve experiments performed in controlled laboratory settings using cells or tissues. It is important to note that these products are not categorized as medicines or drugs, and they have not received approval from the FDA for the prevention, treatment, or cure of any medical condition, ailment, or disease. We must emphasize that any form of bodily introduction of these products into humans or animals is strictly prohibited by law. It is essential to adhere to these guidelines to ensure compliance with legal and ethical standards in research and experimentation.