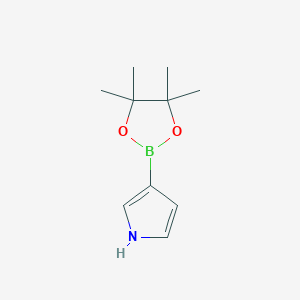
3-(4,4,5,5-Tetramethyl-1,3,2-dioxaborolan-2-YL)-1H-pyrrole
Overview
Description
3-(4,4,5,5-Tetramethyl-1,3,2-dioxaborolan-2-YL)-1H-pyrrole (CAS: 214360-77-7) is a boron-containing heterocyclic compound with the molecular formula C₁₀H₁₆BNO₂ and a molecular weight of 193.05 g/mol . It features a pyrrole ring substituted with a pinacol boronate ester group at the 3-position, making it a valuable intermediate in Suzuki-Miyaura cross-coupling reactions for synthesizing biaryls, pharmaceuticals, and optoelectronic materials . The compound is sensitive to moisture and oxygen, requiring storage under inert atmospheres at -20°C .
Biological Activity
3-(4,4,5,5-Tetramethyl-1,3,2-dioxaborolan-2-YL)-1H-pyrrole is a compound of significant interest in medicinal chemistry and biological research due to its unique structural features and potential therapeutic applications. This article provides a detailed overview of its biological activity, including its synthesis, mechanisms of action, and relevant case studies.
Structural Overview
The compound features a pyrrole ring substituted with a dioxaborolane moiety. This structural configuration enhances its reactivity and interaction with biological targets. The presence of the boron atom in the dioxaborolane contributes to its ability to form reversible covalent bonds with biomolecules, making it a valuable candidate for drug development.
Mechanisms of Biological Activity
-
Enzyme Inhibition :
- The compound has been shown to inhibit specific enzymes involved in various biological pathways. For instance, it acts as an inhibitor of DYRK1A (Dual-specificity Tyrosine-Phosphorylation-Regulated Kinase 1A), which is implicated in neurodegenerative diseases such as Alzheimer's disease. In vitro studies demonstrated that derivatives of this compound exhibited nanomolar-level inhibitory activity against DYRK1A, highlighting their potential in treating cognitive decline associated with neurodegeneration .
- Antioxidant Properties :
- Anti-inflammatory Effects :
Case Study 1: DYRK1A Inhibition
Case Study 2: Antioxidant Activity
Applications in Drug Development
The unique properties of this compound make it a promising candidate for various applications:
- Drug Design : Its ability to selectively inhibit specific kinases positions it as a lead compound for developing treatments for diseases like Alzheimer's and cancer.
- Biological Imaging : The fluorescent properties of boron-containing compounds allow their use as probes for imaging cellular processes .
Summary Table of Biological Activities
Scientific Research Applications
Organic Synthesis
Versatile Building Block
This compound serves as a crucial building block in organic chemistry. Its boron-containing structure allows for the formation of carbon-boron bonds, facilitating the synthesis of complex organic molecules. It is particularly useful in the development of pharmaceuticals and agrochemicals where precision in molecular construction is essential.
Reaction Enhancements
The presence of the dioxaborolane moiety enhances reactivity and selectivity in various coupling reactions, such as Suzuki-Miyaura cross-coupling reactions. This property makes it invaluable for synthesizing biologically active compounds and fine chemicals .
Biological Imaging
Fluorescent Probes
The unique structural characteristics of 3-(4,4,5,5-Tetramethyl-1,3,2-dioxaborolan-2-YL)-1H-pyrrole enable its use in the design of fluorescent probes. These probes are critical for biological imaging applications, allowing researchers to visualize cellular processes in real time. The compound's ability to emit fluorescence upon excitation makes it suitable for tracking biological interactions and cellular dynamics .
Drug Development
Targeted Drug Design
In drug development, this compound plays a role in designing new drug candidates that can target specific biological pathways. Its boron atom can interact with various biomolecules, enhancing the efficacy and specificity of potential therapeutic agents. This application is particularly relevant in oncology and infectious disease research where targeted therapies are paramount .
Stability and Bioavailability
Compounds containing boron often exhibit improved stability and bioavailability compared to their non-boron counterparts. This characteristic is beneficial for developing drugs that require prolonged action or reduced side effects .
Materials Science
Advanced Materials Development
In materials science, this compound is utilized in creating advanced materials such as polymers and nanomaterials. These materials can be applied in electronics and coatings where enhanced performance and durability are desired .
Nanotechnology Applications
The compound's properties make it suitable for applications in nanotechnology, including the development of nanoscale devices and sensors that require precise control over chemical interactions at the molecular level .
Summary Table of Applications
Application Area | Description |
---|---|
Organic Synthesis | Serves as a building block for complex organic molecules; enhances reaction yields. |
Biological Imaging | Used to create fluorescent probes for real-time visualization of cellular processes. |
Drug Development | Aids in designing targeted therapies with improved stability and bioavailability. |
Materials Science | Applied in developing advanced materials for electronics and coatings with enhanced properties. |
Case Study 1: Fluorescent Probes
A study demonstrated the use of this compound as a fluorescent probe for imaging cancer cells. The probe showed high specificity and sensitivity towards tumor markers, allowing for effective monitoring of cancer progression.
Case Study 2: Drug Development
Research involving this compound highlighted its role in synthesizing a new class of anti-cancer drugs that target specific pathways involved in tumor growth. The results indicated a significant reduction in tumor size in preclinical models.
Case Study 3: Material Applications
In materials science research, the incorporation of this compound into polymer matrices resulted in enhanced mechanical properties and thermal stability. This advancement has implications for developing next-generation electronic devices.
Q & A
Basic Research Questions
Q. What established synthetic methodologies are used to prepare 3-(4,4,5,5-tetramethyl-1,3,2-dioxaborolan-2-yl)-1H-pyrrole?
- Methodology : The compound is typically synthesized via palladium-catalyzed borylation of pyrrole derivatives. For example, a multi-step procedure involves coupling a pyrrole precursor with a boronic ester under inert conditions. A key step includes using Pd catalysts (e.g., PdCl₂(dppf)) and ligands to facilitate boron-pyrrole bond formation. Purification often employs column chromatography with hexanes/ethyl acetate and triethylamine to mitigate boronic ester hydrolysis .
- Critical Parameters : Reaction temperature (60–80°C), anhydrous solvents (THF or DME), and stoichiometric control of the boronic ester precursor.
Q. What analytical techniques confirm the structural integrity and purity of this compound?
- Primary Methods :
- NMR Spectroscopy : ¹H NMR identifies pyrrole protons (δ ~6.5–7.0 ppm), while ¹¹B NMR confirms the boronic ester moiety (δ ~30 ppm) .
- X-ray Crystallography : Resolves molecular geometry, including bond lengths and angles of the dioxaborolane ring and pyrrole .
- HPLC : Quantifies purity (>97%) and detects hydrolyzed byproducts (e.g., boronic acid) .
Q. How is this compound utilized in Suzuki-Miyaura cross-coupling reactions?
- Protocol : The boronic ester acts as a coupling partner with aryl/heteroaryl halides. A standard reaction uses Pd(PPh₃)₄ (2–5 mol%), Na₂CO₃ (2 equiv), and a DME/H₂O solvent system. For electron-deficient aryl halides, adding SPhos ligand improves efficiency .
- Key Considerations : Moisture sensitivity necessitates anhydrous conditions; inert atmosphere (N₂/Ar) prevents boronic ester decomposition.
Q. What storage and handling practices ensure compound stability?
- Best Practices :
- Store at –20°C under inert gas (argon) in amber vials to prevent light-/moisture-induced hydrolysis.
- Use freshly distilled solvents (e.g., THF) during synthesis to avoid trace water .
Advanced Research Questions
Q. How can contradictory reports on coupling efficiency with electron-deficient aryl halides be resolved?
- Methodological Approach :
- Kinetic Studies : Monitor reaction progress via in-situ ¹H NMR to identify rate-limiting steps.
- Ligand Screening : Test bulky ligands (e.g., DavePhos) to stabilize Pd intermediates and reduce protodeboronation .
- Base Optimization : Replace Na₂CO₃ with CsF to enhance solubility in polar aprotic solvents (e.g., DMF) .
Q. What computational tools predict regioselectivity in transition-metal-catalyzed reactions involving this compound?
- Strategy :
- DFT Calculations : Model transition states to evaluate steric/electronic effects of the pyrrole NH group on coupling sites.
- Hammett Analysis : Correlate substituent effects on the pyrrole ring with reaction rates .
Q. How does the pyrrole NH group influence boronic ester stability under acidic/basic conditions?
- Experimental Design :
- Comparative Studies : Synthesize NH-protected analogs (e.g., Boc-pyrrole) and compare hydrolysis rates via ¹¹B NMR.
- pH-Dependent Stability Assays : Expose the compound to buffered solutions (pH 3–10) and monitor degradation .
Q. What emerging applications exist for this compound in medicinal chemistry?
- Case Studies :
- Anticancer Agents : Serve as a boron carrier in boron neutron capture therapy (BNCT) due to tumor-targeting potential .
- Proteolysis-Targeting Chimeras (PROTACs) : Linker for assembling bifunctional molecules via Suzuki coupling .
Q. How can reaction yields be improved in large-scale syntheses?
- Optimization Strategies :
- Continuous Flow Reactors : Enhance heat/mass transfer and reduce side reactions (e.g., dimerization) .
- Microwave-Assisted Synthesis : Accelerate borylation steps while maintaining high regioselectivity .
Q. What structural modifications enhance the compound’s reactivity in C–H borylation?
Comparison with Similar Compounds
The following analysis compares 3-(4,4,5,5-tetramethyl-1,3,2-dioxaborolan-2-YL)-1H-pyrrole with structurally related boronate esters, focusing on reactivity, physical properties, and applications.
Structural Analogs and Reactivity
Limitations and Challenges
Preparation Methods
Direct Borylation of Pyrrole Derivatives via Transition Metal Catalysis
A common and efficient approach to prepare 3-(4,4,5,5-tetramethyl-1,3,2-dioxaborolan-2-yl)-1H-pyrrole involves the direct borylation of pyrrole or pyrrole derivatives using bis(pinacolato)diboron under transition metal catalysis, often iridium-based catalysts.
-
- Iridium complexes such as bis(1,5-cyclooctadiene)di-μ-methoxydiiridium(I) dimer combined with di-tert-butylbipyridine ligands are employed.
- Reaction typically conducted in tetrahydrofuran (THF) or cyclohexane.
- Temperature range: 70–90 °C.
- Reaction time: 16–48 hours under inert atmosphere (nitrogen).
-
- The iridium catalyst activates the C–H bond at the 3-position of pyrrole, facilitating the formation of the C–B bond with bis(pinacolato)diboron.
Lithiation Followed by Electrophilic Borylation
Another established method involves lithiation of the pyrrole ring followed by quenching with a boron electrophile to install the boronate ester.
-
- Pyrrole is treated with a strong base such as n-butyllithium (n-BuLi) or tert-butyllithium (t-BuLi) at low temperature (0 °C) to generate the lithiated intermediate at the 3-position.
- This intermediate is then reacted with a boron electrophile, such as 2-(4-(bromomethyl)phenyl)-1,3,2-dioxaborolane or trimethylborate.
- The reaction mixture is stirred at elevated temperatures (e.g., 65 °C) for several hours.
- Workup involves aqueous quenching and extraction, followed by purification via preparative thin-layer chromatography or column chromatography.
-
- Moderate yields around 34–69% have been reported.
- The boronate group is often protected as a pinacol ester to improve stability during isolation and purification.
Data Table: Summary of Preparation Methods
Method | Key Reagents & Catalysts | Conditions | Yield Range | Notes |
---|---|---|---|---|
Transition Metal-Catalyzed C–H Borylation | Bis(pinacolato)diboron, Iridium catalyst, dtbpy ligand | 70–90 °C, 16–48 h, THF or cyclohexane | ~70% | Direct borylation at pyrrole 3-position |
Lithiation + Electrophilic Borylation | Pyrrole, n-BuLi or t-BuLi, boron electrophile (e.g., bromomethyl dioxaborolane) | 0 °C lithiation, then 65 °C reaction | 34–69% | Requires careful handling of organolithium |
Late-Stage Boronate Introduction | Halogenated pyrrole, t-BuLi, trimethylborate, pinacol | Lithiation at low temp, acid hydrolysis | Moderate | Avoids early boronate instability |
Paal-Knorr + Suzuki Coupling | 1,4-dicarbonyl compounds, sulfonyl chloride, Pd catalyst, bis(pinacolato)diboron | Pd-catalyzed coupling, inert atmosphere | Variable | Suitable for functionalized pyrrole derivatives |
Research Findings and Notes
Stability Considerations: The boronate group is typically protected as a pinacol ester to improve stability and facilitate purification, as unprotected boronic acids are prone to polymerization and hydrolysis.
Purification Techniques: Preparative thin-layer chromatography (PTLC) and silica gel column chromatography are commonly used to isolate pure products. Use of Dean–Stark apparatus during esterification ensures removal of water to drive equilibrium toward ester formation.
Analytical Confirmation: Characterization of the synthesized compounds is typically done using proton nuclear magnetic resonance (^1H NMR), carbon NMR (^13C NMR), and mass spectrometry (MS). Reported NMR shifts and mass fragments confirm the structure and purity of the boronate ester-substituted pyrroles.
Industrial Scale-Up: While literature primarily reports lab-scale syntheses, industrial production would likely optimize these methods using continuous flow reactors, automated reagent addition, and chromatographic purification to enhance yield and reproducibility.
Properties
IUPAC Name |
3-(4,4,5,5-tetramethyl-1,3,2-dioxaborolan-2-yl)-1H-pyrrole | |
---|---|---|
Source | PubChem | |
URL | https://pubchem.ncbi.nlm.nih.gov | |
Description | Data deposited in or computed by PubChem | |
InChI |
InChI=1S/C10H16BNO2/c1-9(2)10(3,4)14-11(13-9)8-5-6-12-7-8/h5-7,12H,1-4H3 | |
Source | PubChem | |
URL | https://pubchem.ncbi.nlm.nih.gov | |
Description | Data deposited in or computed by PubChem | |
InChI Key |
VLOXXHSBLUSPCW-UHFFFAOYSA-N | |
Source | PubChem | |
URL | https://pubchem.ncbi.nlm.nih.gov | |
Description | Data deposited in or computed by PubChem | |
Canonical SMILES |
B1(OC(C(O1)(C)C)(C)C)C2=CNC=C2 | |
Source | PubChem | |
URL | https://pubchem.ncbi.nlm.nih.gov | |
Description | Data deposited in or computed by PubChem | |
Molecular Formula |
C10H16BNO2 | |
Source | PubChem | |
URL | https://pubchem.ncbi.nlm.nih.gov | |
Description | Data deposited in or computed by PubChem | |
DSSTOX Substance ID |
DTXSID50676991 | |
Record name | 3-(4,4,5,5-Tetramethyl-1,3,2-dioxaborolan-2-yl)-1H-pyrrole | |
Source | EPA DSSTox | |
URL | https://comptox.epa.gov/dashboard/DTXSID50676991 | |
Description | DSSTox provides a high quality public chemistry resource for supporting improved predictive toxicology. | |
Molecular Weight |
193.05 g/mol | |
Source | PubChem | |
URL | https://pubchem.ncbi.nlm.nih.gov | |
Description | Data deposited in or computed by PubChem | |
CAS No. |
214360-77-7 | |
Record name | 3-(4,4,5,5-Tetramethyl-1,3,2-dioxaborolan-2-yl)-1H-pyrrole | |
Source | EPA DSSTox | |
URL | https://comptox.epa.gov/dashboard/DTXSID50676991 | |
Description | DSSTox provides a high quality public chemistry resource for supporting improved predictive toxicology. | |
Record name | 3-(tetramethyl-1,3,2-dioxaborolan-2-yl)-1H-pyrrole | |
Source | European Chemicals Agency (ECHA) | |
URL | https://echa.europa.eu/information-on-chemicals | |
Description | The European Chemicals Agency (ECHA) is an agency of the European Union which is the driving force among regulatory authorities in implementing the EU's groundbreaking chemicals legislation for the benefit of human health and the environment as well as for innovation and competitiveness. | |
Explanation | Use of the information, documents and data from the ECHA website is subject to the terms and conditions of this Legal Notice, and subject to other binding limitations provided for under applicable law, the information, documents and data made available on the ECHA website may be reproduced, distributed and/or used, totally or in part, for non-commercial purposes provided that ECHA is acknowledged as the source: "Source: European Chemicals Agency, http://echa.europa.eu/". Such acknowledgement must be included in each copy of the material. ECHA permits and encourages organisations and individuals to create links to the ECHA website under the following cumulative conditions: Links can only be made to webpages that provide a link to the Legal Notice page. | |
Retrosynthesis Analysis
AI-Powered Synthesis Planning: Our tool employs the Template_relevance Pistachio, Template_relevance Bkms_metabolic, Template_relevance Pistachio_ringbreaker, Template_relevance Reaxys, Template_relevance Reaxys_biocatalysis model, leveraging a vast database of chemical reactions to predict feasible synthetic routes.
One-Step Synthesis Focus: Specifically designed for one-step synthesis, it provides concise and direct routes for your target compounds, streamlining the synthesis process.
Accurate Predictions: Utilizing the extensive PISTACHIO, BKMS_METABOLIC, PISTACHIO_RINGBREAKER, REAXYS, REAXYS_BIOCATALYSIS database, our tool offers high-accuracy predictions, reflecting the latest in chemical research and data.
Strategy Settings
Precursor scoring | Relevance Heuristic |
---|---|
Min. plausibility | 0.01 |
Model | Template_relevance |
Template Set | Pistachio/Bkms_metabolic/Pistachio_ringbreaker/Reaxys/Reaxys_biocatalysis |
Top-N result to add to graph | 6 |
Feasible Synthetic Routes
Disclaimer and Information on In-Vitro Research Products
Please be aware that all articles and product information presented on BenchChem are intended solely for informational purposes. The products available for purchase on BenchChem are specifically designed for in-vitro studies, which are conducted outside of living organisms. In-vitro studies, derived from the Latin term "in glass," involve experiments performed in controlled laboratory settings using cells or tissues. It is important to note that these products are not categorized as medicines or drugs, and they have not received approval from the FDA for the prevention, treatment, or cure of any medical condition, ailment, or disease. We must emphasize that any form of bodily introduction of these products into humans or animals is strictly prohibited by law. It is essential to adhere to these guidelines to ensure compliance with legal and ethical standards in research and experimentation.