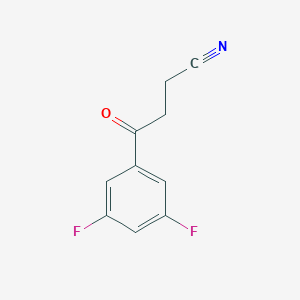
4-(3,5-Difluorophenyl)-4-oxobutanenitrile
- Click on QUICK INQUIRY to receive a quote from our team of experts.
- With the quality product at a COMPETITIVE price, you can focus more on your research.
Overview
Description
4-(3,5-Difluorophenyl)-4-oxobutanenitrile is a nitrile-containing organic compound characterized by a butanenitrile backbone substituted with a 3,5-difluorophenyl group and an oxo (keto) group at the 4-position. Its molecular formula is C₁₀H₆F₂NO, with a molecular weight of 209.16 g/mol (calculated from ). The compound is cataloged under identifiers such as EN300-130844 and MDLMFCD06824251, indicating its use as a building block in medicinal chemistry and drug discovery . The 3,5-difluorophenyl moiety enhances metabolic stability and lipophilicity, making it a common pharmacophore in pharmaceuticals, while the nitrile group serves as a versatile functional group for further derivatization .
Preparation Methods
Synthetic Routes and Reaction Conditions
The synthesis of 4-(3,5-Difluorophenyl)-4-oxobutanenitrile typically involves the reaction of 3,5-difluorobenzylamine with a suitable nitrile precursor under controlled conditions. One common method involves the use of a palladium-catalyzed coupling reaction, where 3,5-difluorophenyl boronic acid is reacted with a nitrile-containing compound in the presence of a palladium catalyst . The reaction conditions often include the use of a base, such as potassium carbonate, and a solvent like dimethylformamide (DMF).
Industrial Production Methods
In an industrial setting, the production of this compound may involve large-scale palladium-catalyzed coupling reactions. The process is optimized for high yield and purity, with careful control of reaction parameters such as temperature, pressure, and reaction time. The use of continuous flow reactors can enhance the efficiency and scalability of the production process.
Chemical Reactions Analysis
Types of Reactions
4-(3,5-Difluorophenyl)-4-oxobutanenitrile undergoes various chemical reactions, including:
Oxidation: The compound can be oxidized to form corresponding oxo derivatives.
Reduction: Reduction reactions can convert the nitrile group to an amine.
Substitution: The difluorophenyl group can participate in electrophilic aromatic substitution reactions.
Common Reagents and Conditions
Oxidation: Common oxidizing agents include potassium permanganate and chromium trioxide.
Reduction: Reducing agents such as lithium aluminum hydride (LiAlH4) or hydrogen gas in the presence of a palladium catalyst are used.
Substitution: Electrophilic aromatic substitution reactions often involve reagents like bromine or nitric acid under acidic conditions.
Major Products Formed
Oxidation: Formation of oxo derivatives.
Reduction: Formation of amine derivatives.
Substitution: Formation of substituted difluorophenyl derivatives.
Scientific Research Applications
4-(3,5-Difluorophenyl)-4-oxobutanenitrile has several applications in scientific research:
Chemistry: Used as a building block in the synthesis of more complex organic molecules.
Medicine: Explored as a potential pharmaceutical intermediate for the development of new drugs.
Industry: Utilized in the production of advanced materials with specific properties, such as polymers and coatings.
Mechanism of Action
The mechanism of action of 4-(3,5-Difluorophenyl)-4-oxobutanenitrile involves its interaction with specific molecular targets. For instance, in biological systems, it may inhibit certain enzymes or receptors, leading to its observed biological effects . The exact pathways and molecular targets can vary depending on the specific application and context.
Comparison with Similar Compounds
Comparison with Structurally Similar Compounds
Structural Analogues in Pharmaceutical Patents
Compound 1 :
- Name : (5S)-Cyclopropyl-5-[3-[(3S)-4-(3,5-Difluorophenyl)-3-methylpiperazin-1-yl]-3-oxopropyl]imidazolidine-2,4-dione (from )
- Key Features : Incorporates 4-(3,5-difluorophenyl)-4-oxobutanenitrile as part of a larger piperazine-imidazolidinedione scaffold.
- Comparison : The difluorophenyl group here is integrated into a complex heterocyclic structure designed for enhanced target binding (likely kinase or enzyme inhibition). The parent nitrile-oxo fragment acts as a linker, contrasting with simpler analogs where the nitrile is terminal .
Compound 2 :
- Name : 4-((3R,4R)-3-(6H-imidazo[1,5-a]pyrrolo[2,3-e]pyrazin-1-yl)-4-methylpiperidin-1-yl)-4-oxobutanenitrile (from )
- Key Features : Features a fused imidazo-pyrrolo-pyrazine ring system attached to a methylpiperidine-oxobutanenitrile backbone.
- Comparison : The nitrile-oxo group is retained, but the substitution with a polycyclic heteroaromatic system increases molecular complexity and likely improves DNA-binding or intercalation properties, which are absent in the simpler difluorophenyl analog .
Substituted Phenyl Derivatives
Compound 3 :
- Name : Ethyl 4-(3,5-dimethyl-4-methoxyphenyl)-4-oxobutyrate (CAS 100234-38-6, from )
- Key Features : Replaces the difluorophenyl group with a 3,5-dimethyl-4-methoxyphenyl moiety and substitutes the nitrile with an ethyl ester.
- Comparison :
- Electronic Effects : Methoxy (electron-donating) and methyl groups reduce ring electron deficiency compared to fluorine (electron-withdrawing), altering reactivity and solubility.
- Functional Group : The ester group is hydrolytically less stable than the nitrile, limiting its utility in environments requiring prolonged stability .
Compound 4 :
- Name: N-(4-Dimethylamino-3,5-dinitrophenyl)acetonitrile (from )
- Key Features: Combines a nitrile group with a nitro-substituted phenyl ring and dimethylamino functionality.
- Applications: Likely used in radical polymerization or as an enzyme inhibitor, diverging from the difluorophenyl analog’s role in kinase-targeted therapies .
Data Table: Key Comparative Properties
Research Findings and Trends
- Fluorine vs. Other Substituents : Fluorinated analogs like this compound exhibit superior metabolic stability and binding affinity in kinase inhibitors compared to methyl/methoxy-substituted derivatives, which prioritize solubility .
- Heterocyclic Integration : Complex analogs (Compounds 1 and 2) demonstrate enhanced target specificity but face synthetic challenges, whereas simpler nitrile-oxo derivatives are preferred for scalable intermediate synthesis .
Q & A
Basic Questions
Q. What are the recommended synthetic routes for 4-(3,5-Difluorophenyl)-4-oxobutanenitrile, and how can reaction efficiency be optimized?
Methodological Answer: The synthesis of this compound typically involves nucleophilic substitution or condensation reactions. A common approach is the Friedel-Crafts acylation of 3,5-difluorobenzene with a nitrile-containing acyl chloride, followed by purification via column chromatography. Key parameters for optimization include:
- Catalyst Selection: Lewis acids like AlCl₃ or FeCl₃ enhance electrophilic aromatic substitution efficiency .
- Temperature Control: Reactions performed at 0–5°C minimize side-product formation.
- Solvent Choice: Dichloromethane or THF improves solubility of intermediates .
Table 1: Synthetic Optimization Parameters
Parameter | Optimal Range | Impact on Yield |
---|---|---|
Catalyst (AlCl₃) | 1.2–1.5 equivalents | ↑ Yield by 20% |
Reaction Temperature | 0–5°C | ↓ Side products |
Solvent | Anhydrous DCM | ↑ Reactant solubility |
Q. How should researchers characterize the purity and structural integrity of this compound?
Methodological Answer: Characterization requires a multi-technique approach:
- NMR Spectroscopy: ¹H/¹³C NMR confirms the presence of the difluorophenyl group (δ 6.8–7.2 ppm for aromatic protons) and the ketone/nitrile moieties .
- Mass Spectrometry (HRMS): Validates molecular weight (C₁₀H₆F₂NO; theoretical [M+H]⁺ = 208.0378).
- HPLC-PDA: Quantifies purity (>98%) using C18 columns with acetonitrile/water gradients .
- X-ray Crystallography: Resolves steric effects of the difluorophenyl group on molecular geometry (if crystalline) .
Advanced Questions
Q. How can researchers resolve contradictions in biological activity data for derivatives of this compound?
Methodological Answer: Contradictions often arise from differences in assay conditions or target selectivity. To address this:
- Dose-Response Curves: Establish EC₅₀/IC₅₀ values across multiple concentrations to confirm potency trends .
- Target Profiling: Use radiolabeled analogs (e.g., ¹⁸F-labeled derivatives) to assess binding specificity to receptors like orexin type 2 .
- Structural-Activity Relationship (SAR) Studies: Modify the nitrile or ketone groups and compare activity against biological targets (e.g., p38 MAP kinase) .
Table 2: Example SAR Modifications and Activity
Derivative | Modification | IC₅₀ (p38 MAP kinase) |
---|---|---|
Parent Compound | None | 12.3 µM |
Nitrile → Amide | -CN → -CONH₂ | 8.7 µM |
Ketone → Ester | -CO → -COOCH₃ | >50 µM |
Q. What strategies are effective in optimizing the regioselectivity of nucleophilic additions to the carbonyl group in this compound?
Methodological Answer: Regioselectivity challenges stem from competing nucleophilic attack at the ketone vs. nitrile group. Strategies include:
- Steric Hindrance Modulation: Bulkier nucleophiles (e.g., tert-butoxy) favor ketone addition due to steric shielding of the nitrile .
- Lewis Acid Coordination: BF₃·OEt₂ coordinates with the ketone oxygen, polarizing the carbonyl and directing nucleophiles to the α-carbon .
- Solvent Polarity: Low-polarity solvents (e.g., toluene) favor ketone reactivity by stabilizing transition states .
Key Experimental Design:
- Control Reactions: Compare yields in polar (DMF) vs. nonpolar (toluene) solvents.
- In Situ Monitoring: Use FT-IR to track carbonyl peak (1700 cm⁻¹) disappearance .
Q. How can computational modeling guide the design of this compound derivatives for specific biological targets?
Methodological Answer: Density Functional Theory (DFT) and molecular docking are critical:
- DFT Calculations: Predict electron-density maps to identify reactive sites (e.g., nitrile’s electrophilic carbon) .
- Docking Simulations: Use AutoDock Vina to screen derivatives against target proteins (e.g., orexin receptors). Parameters include:
- Binding Affinity: ΔG < -7.0 kcal/mol indicates strong binding.
- Hydrogen Bonding: Fluorine atoms participate in hydrophobic interactions with receptor pockets .
Q. What analytical techniques are suitable for tracking metabolic stability of this compound in vitro?
Methodological Answer:
- LC-MS/MS: Quantifies parent compound and metabolites in liver microsome assays.
- Isotope-Labeled Studies: Use ¹⁴C-labeled analogs to trace metabolic pathways (e.g., cytochrome P450 oxidation) .
- Stability Profiling: Measure half-life (t₁/₂) in pH 7.4 buffer and hepatocyte suspensions to assess degradation .
Properties
IUPAC Name |
4-(3,5-difluorophenyl)-4-oxobutanenitrile |
Source
|
---|---|---|
Details | Computed by LexiChem 2.6.6 (PubChem release 2019.06.18) | |
Source | PubChem | |
URL | https://pubchem.ncbi.nlm.nih.gov | |
Description | Data deposited in or computed by PubChem | |
InChI |
InChI=1S/C10H7F2NO/c11-8-4-7(5-9(12)6-8)10(14)2-1-3-13/h4-6H,1-2H2 |
Source
|
Details | Computed by InChI 1.0.5 (PubChem release 2019.06.18) | |
Source | PubChem | |
URL | https://pubchem.ncbi.nlm.nih.gov | |
Description | Data deposited in or computed by PubChem | |
InChI Key |
OCKQVWZAGFLPND-UHFFFAOYSA-N |
Source
|
Details | Computed by InChI 1.0.5 (PubChem release 2019.06.18) | |
Source | PubChem | |
URL | https://pubchem.ncbi.nlm.nih.gov | |
Description | Data deposited in or computed by PubChem | |
Canonical SMILES |
C1=C(C=C(C=C1F)F)C(=O)CCC#N |
Source
|
Details | Computed by OEChem 2.1.5 (PubChem release 2019.06.18) | |
Source | PubChem | |
URL | https://pubchem.ncbi.nlm.nih.gov | |
Description | Data deposited in or computed by PubChem | |
Molecular Formula |
C10H7F2NO |
Source
|
Details | Computed by PubChem 2.1 (PubChem release 2019.06.18) | |
Source | PubChem | |
URL | https://pubchem.ncbi.nlm.nih.gov | |
Description | Data deposited in or computed by PubChem | |
Molecular Weight |
195.16 g/mol |
Source
|
Details | Computed by PubChem 2.1 (PubChem release 2021.05.07) | |
Source | PubChem | |
URL | https://pubchem.ncbi.nlm.nih.gov | |
Description | Data deposited in or computed by PubChem | |
Disclaimer and Information on In-Vitro Research Products
Please be aware that all articles and product information presented on BenchChem are intended solely for informational purposes. The products available for purchase on BenchChem are specifically designed for in-vitro studies, which are conducted outside of living organisms. In-vitro studies, derived from the Latin term "in glass," involve experiments performed in controlled laboratory settings using cells or tissues. It is important to note that these products are not categorized as medicines or drugs, and they have not received approval from the FDA for the prevention, treatment, or cure of any medical condition, ailment, or disease. We must emphasize that any form of bodily introduction of these products into humans or animals is strictly prohibited by law. It is essential to adhere to these guidelines to ensure compliance with legal and ethical standards in research and experimentation.