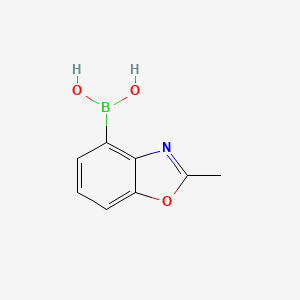
(2-methyl-1,3-benzoxazol-4-yl)boronic acid
- Click on QUICK INQUIRY to receive a quote from our team of experts.
- With the quality product at a COMPETITIVE price, you can focus more on your research.
Overview
Description
(2-Methyl-1,3-benzoxazol-4-yl)boronic acid is an aromatic organoboron compound featuring a benzoxazole core substituted with a methyl group at position 2 and a boronic acid moiety at position 4. Benzoxazole derivatives are known for their electron-deficient aromatic systems, which can modulate the electronic properties of appended functional groups, including boronic acids. Boronic acids are widely utilized in medicinal chemistry, materials science, and catalysis due to their reversible diol-binding capacity and ability to participate in Suzuki-Miyaura cross-coupling reactions .
Preparation Methods
Synthetic Routes and Reaction Conditions
The synthesis of (2-methyl-1,3-benzoxazol-4-yl)boronic acid typically involves the reaction of 2-methylbenzoxazole with a boron-containing reagent. One common method is the Suzuki-Miyaura coupling reaction, which involves the palladium-catalyzed cross-coupling of an aryl halide with a boronic acid or boronate ester . This reaction is known for its mild conditions and high functional group tolerance, making it an ideal choice for the synthesis of this compound.
Industrial Production Methods
Industrial production of this compound may involve large-scale Suzuki-Miyaura coupling reactions, utilizing efficient catalysts and optimized reaction conditions to maximize yield and minimize costs. The use of continuous flow reactors and automated systems can further enhance the efficiency and scalability of the production process .
Chemical Reactions Analysis
Cross-Coupling Reactions
The compound participates in Suzuki-Miyaura coupling , a widely used method for forming carbon-carbon bonds. For example, coupling with aryl halides or triflates under palladium catalysis (e.g., Pd(PPh₃)₄) in a mixed solvent system (e.g., ethanol/toluene) yields biaryl derivatives . Microwave-assisted conditions (160°C, 30 min) have also been reported for efficient coupling .
Representative reaction :
-
Reaction partners : Aryl halides (e.g., 4-formylphenylboronic acid)
-
Catalyst : Pd(PPh₃)₄ or CuI with 8-quinolinol
Reactant | Catalyst | Solvent | Yield |
---|---|---|---|
4-formylphenylboronic acid | Pd(PPh₃)₄ | EtOH/toluene | 82% |
4-propylpyrimidinylboronic acid | CuI/8-quinolinol | DMSO | 37% |
Reactivity Trends
The benzoxazole scaffold influences reactivity:
-
Electron-withdrawing groups (e.g., nitro) on the benzoxazole ring reduce reaction efficiency .
-
Electron-donating groups (e.g., methoxy) may also decrease yields due to steric effects .
-
Heterocyclic partners (e.g., pyrimidine, quinoline) show varied compatibility, with yields ranging from 37% to 92% .
Substituent | Position | Yield (Suzuki coupling) |
---|---|---|
H | Para | 92% |
CF₃ | Para | 55% |
CN | Para | 37% |
Medicinal Chemistry
-
Drug discovery : Benzoxazole derivatives show promise in targeting enzymes (e.g., acid ceramidase) .
-
Bioactivity : Potential antimicrobial and antipathogenic properties linked to boronic acid functionality.
Structural and Analytical Data
Key spectroscopic features :
Property | Value |
---|---|
Molecular weight | 211.06 g/mol |
Solubility | Insoluble in H₂O |
Scientific Research Applications
(2-Methyl-1,3-benzoxazol-4-yl)boronic acid has a wide range of applications in scientific research:
Biology: The compound can be used in the development of boron-containing drugs and as a tool for studying biological processes involving boron.
Mechanism of Action
The mechanism of action of (2-methyl-1,3-benzoxazol-4-yl)boronic acid involves its ability to form reversible covalent bonds with diols and other nucleophilic groups. This property is particularly useful in molecular recognition and catalysis, where the boronic acid group can interact with specific molecular targets and facilitate various chemical transformations .
Comparison with Similar Compounds
Structural Analogs and Electronic Effects
a. Heterocyclic Boronic Acids
- Triazole-substituted Boronic Acids : 1-Amido-2-triazolylethaneboronic acid (e.g., MB076 derivatives) replaces phenyl rings with triazole moieties. This substitution maintains inhibitory activity against β-lactamases (Ki ~ nM range) while improving in vitro efficacy (MIC values) compared to phenyl analogs . The benzoxazole group in the target compound may similarly enhance target selectivity due to its planar, electron-deficient structure.
- Pyrazole Boronic Acids : Pyrazole-based analogs (e.g., 3-methyl-1H-pyrazol-4-yl boronic acid) exhibit structural similarity (Tanimoto coefficient ≥0.84) but differ in ring hybridization and electronic effects. Pyrazole’s sp²-hybridized nitrogen atoms may reduce steric hindrance compared to benzoxazole’s fused oxygen and nitrogen atoms .
b. Borinic Acids (R₂B(OH))
Borinic acids, such as diphenylborinic acid, exhibit ~10-fold higher association constants for diols (e.g., catechol) than boronic acids. This enhanced binding is attributed to reduced steric bulk and altered Lewis acidity. However, boronic acids like the target compound remain preferable for physiological applications due to their hydrolytic stability and broader synthetic accessibility .
pKa and Reactivity
- pKa Trends : The benzoxazole ring’s electron-withdrawing nature likely lowers the pKa of (2-methyl-1,3-benzoxazol-4-yl)boronic acid compared to phenyl boronic acids (pKa ~8.6–9.2). For example, fluoro-substituted boronic acids (pKa ~7.5–8.0) demonstrate that electron-withdrawing groups stabilize the boronate conjugate base, enhancing diol-binding at physiological pH .
- Glucose Binding : Boronic acids like 3-AcPBA and 4-MCPBA exhibit low glucose association constants (Ka ~10² M⁻¹) due to high pKa (>9). In contrast, benzoxazole-derived boronic acids may achieve Ka >10³ M⁻¹ if their pKa is closer to physiological pH (7.4), enabling applications in glucose sensing .
Data Tables
Table 1: pKa and Binding Properties of Selected Boronic Acids
Q & A
Basic Research Questions
Q. What are the optimal synthetic routes for (2-methyl-1,3-benzoxazol-4-yl)boronic acid, and how can yield and purity be maximized?
Synthesis of this compound typically involves Suzuki-Miyaura cross-coupling or protodeboronation of boronic esters. Key challenges include minimizing boroxine formation (dehydration/trimerization) and improving selectivity. To enhance yield:
- Use protecting groups (e.g., diols or sugars) to stabilize the boronic acid during synthesis .
- Optimize reaction conditions (e.g., palladium catalysts, base selection, and solvent polarity) to reduce side reactions .
- Purify via non-silica methods (e.g., recrystallization) to avoid irreversible binding with silica gel .
Q. How can researchers address analytical challenges in characterizing this compound?
Boronic acids often complicate mass spectrometry (MS) analysis due to dehydration or boroxine formation. Methodological solutions include:
- Derivatization : Convert to boronic esters using diols (e.g., 2,3-butanedione) to stabilize the compound for MALDI-MS or LC-MS .
- Buffer optimization : Adjust pH and ionic strength to suppress non-specific interactions during chromatography or electrophoresis .
- Thermogravimetric analysis (TGA) : Assess thermal stability to identify decomposition thresholds (e.g., stability up to 600°C for some analogs) .
Table 1 : Common Derivatization Agents for Boronic Acid Stabilization
Agent | Efficiency | Application | Reference |
---|---|---|---|
2,3-Butanedione | High | MALDI-MS | |
Diols (e.g., ethylene glycol) | Moderate | LC-MS | |
Sugars (e.g., mannitol) | High | Structural analysis |
Advanced Research Questions
Q. How can computational methods guide the rational design of this compound derivatives for drug discovery?
- Bioisosteric replacement : Replace carbonyl groups in β-lactams or protease inhibitors with boronic acid moieties to enhance binding affinity .
- Docking studies : Use tools like the Boronic Acid Navigator to predict interactions with biological targets (e.g., enzymes or glycoproteins) .
- QSAR modeling : Correlate substituent effects (e.g., methyl or halogen groups) with bioactivity (e.g., antibacterial or anticancer properties) .
Q. What strategies mitigate non-specific interactions when studying boronic acid-glycoprotein binding?
Secondary interactions (e.g., hydrophobic or electrostatic forces) can reduce selectivity. To improve specificity:
- Buffer modulation : Use high-pH buffers (e.g., Tris-HCl pH 8.5) to enhance boronate-diol ester formation .
- Surface functionalization : Immobilize boronic acids on matrices (e.g., gold nanoparticles) to sterically hinder non-specific binding .
- Competitive assays : Introduce free diols (e.g., sorbitol) to displace weakly bound proteins .
Q. How do structural modifications influence the thermal stability of this compound derivatives?
Thermal degradation pathways depend on substituents:
- Electron-withdrawing groups (e.g., -Cl, -F): Increase stability by reducing boroxine formation .
- Bulkier substituents (e.g., tert-butyl): Sterically hinder trimerization, enhancing thermal resistance .
Table 2 : Thermal Stability of Boronic Acid Derivatives
Q. What are the implications of contradictory data in boronic acid reactivity studies, and how can they be resolved?
Contradictions often arise from:
- Solvent effects : Polar aprotic solvents (e.g., DMF) may accelerate boroxine formation vs. aqueous buffers .
- pH dependency : Reactivity in Suzuki couplings varies with base strength (e.g., K2CO3 vs. CsF) .
- Instrumental artifacts : MALDI-MS may underestimate boronic acid concentration due to dehydration; validate with NMR or derivatized LC-MS .
Resolution workflow :
Replicate experiments under standardized conditions (solvent, pH, temperature).
Cross-validate using orthogonal techniques (e.g., TGA + XRD for crystallinity).
Apply multivariate analysis to isolate critical variables .
Q. Key Takeaways
- Synthesis : Prioritize derivatization and non-silica purification.
- Analysis : Combine MS with stabilizing agents or thermal profiling.
- Design : Leverage computational tools for bioactivity prediction.
- Data conflicts : Systematically isolate experimental variables.
For further reading, consult the Boronic Acid Navigator and structural databases (e.g., Cambridge Structural Database) using SHELX-refined crystallographic data .
Properties
Molecular Formula |
C8H8BNO3 |
---|---|
Molecular Weight |
176.97 g/mol |
IUPAC Name |
(2-methyl-1,3-benzoxazol-4-yl)boronic acid |
InChI |
InChI=1S/C8H8BNO3/c1-5-10-8-6(9(11)12)3-2-4-7(8)13-5/h2-4,11-12H,1H3 |
InChI Key |
ZJVRFUWWBILDOK-UHFFFAOYSA-N |
Canonical SMILES |
B(C1=C2C(=CC=C1)OC(=N2)C)(O)O |
Origin of Product |
United States |
Disclaimer and Information on In-Vitro Research Products
Please be aware that all articles and product information presented on BenchChem are intended solely for informational purposes. The products available for purchase on BenchChem are specifically designed for in-vitro studies, which are conducted outside of living organisms. In-vitro studies, derived from the Latin term "in glass," involve experiments performed in controlled laboratory settings using cells or tissues. It is important to note that these products are not categorized as medicines or drugs, and they have not received approval from the FDA for the prevention, treatment, or cure of any medical condition, ailment, or disease. We must emphasize that any form of bodily introduction of these products into humans or animals is strictly prohibited by law. It is essential to adhere to these guidelines to ensure compliance with legal and ethical standards in research and experimentation.