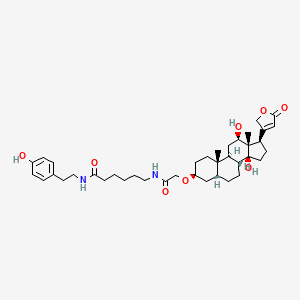
Digoxigenin Tyramide
- Click on QUICK INQUIRY to receive a quote from our team of experts.
- With the quality product at a COMPETITIVE price, you can focus more on your research.
Overview
Description
Digoxigenin tyramide is a critical reagent in molecular biology, particularly for tyramide signal amplification (TSA) in techniques like fluorescence in situ hybridization (FISH) and immunohistochemistry. It consists of digoxigenin (DIG), a steroid hapten derived from Digitalis plants, conjugated to tyramide. The DIG moiety binds specifically to anti-DIG antibodies, while tyramide undergoes peroxidase-catalyzed deposition, enabling localized signal amplification . This system achieves up to 500–1000-fold signal enhancement compared to conventional detection methods, making it indispensable for low-abundance target detection .
Preparation Methods
Synthetic Routes and Reaction Conditions
The synthesis of Digoxigenin Tyramide involves the conjugation of digoxigenin, a steroid hapten, to tyramide. The process typically includes the following steps:
Activation of Digoxigenin: Digoxigenin is activated using a coupling agent such as N-hydroxysuccinimide (NHS) in the presence of a carbodiimide, forming an NHS ester.
Conjugation to Tyramide: The activated digoxigenin is then reacted with tyramide in a suitable solvent, such as dimethylformamide (DMF), under mild conditions to form this compound.
Industrial Production Methods
Industrial production of this compound follows similar synthetic routes but on a larger scale. The process is optimized for high yield and purity, often involving automated synthesis and purification systems to ensure consistency and efficiency .
Chemical Reactions Analysis
Types of Reactions
Digoxigenin Tyramide primarily undergoes the following types of reactions:
Oxidation: Catalyzed by horseradish peroxidase in the presence of hydrogen peroxide, leading to the formation of reactive intermediates.
Covalent Bond Formation: The reactive intermediates form covalent bonds with electron-rich regions of target molecules, facilitating signal amplification.
Common Reagents and Conditions
Horseradish Peroxidase (HRP): Catalyzes the oxidation of tyramide.
Hydrogen Peroxide (H₂O₂): Acts as an oxidizing agent.
Buffer Solutions: Maintain optimal pH for the reactions.
Major Products
The major product of these reactions is the covalently bound this compound on the target molecule, which can be detected using anti-digoxigenin antibodies .
Scientific Research Applications
Digoxigenin Tyramide has a wide range of applications in scientific research:
Chemistry: Used in the detection of specific nucleic acid sequences through in situ hybridization.
Biology: Facilitates the study of gene expression and localization in cells and tissues.
Medicine: Employed in diagnostic techniques to detect pathogens and genetic abnormalities.
Industry: Utilized in the development of biosensors and diagnostic assays
Mechanism of Action
The mechanism of action of Digoxigenin Tyramide involves the following steps:
Hybridization: Digoxigenin-labeled probes hybridize to the target nucleic acid sequences.
Detection: Anti-digoxigenin HRP binds to the digoxigenin-labeled probes.
Signal Amplification: HRP catalyzes the oxidation of tyramide in the presence of hydrogen peroxide, forming reactive intermediates.
Covalent Bond Formation: The reactive intermediates form covalent bonds with nearby molecules, amplifying the signal.
Comparison with Similar Compounds
Comparison with Similar Tyramide Conjugates
Tyramide-based signal amplification systems utilize various haptens or fluorophores. Below is a detailed comparison of digoxigenin tyramide with biotin-, fluorochrome-, and trinitrophenyl (TNP)-tyramides:
Sensitivity and Signal-to-Noise Ratio
- This compound: Highest sensitivity among non-radioactive probes, rivaling radioactive methods when combined with alkaline phosphatase (AP) detection . Minimal background due to the absence of endogenous digoxigenin in mammalian tissues . Example: In BMP2 mRNA detection, DIG-TSA with AP matched the sensitivity of radioactive in situ hybridization .
- Biotin-Tyramide: Lower specificity due to endogenous biotin in tissues (e.g., liver, kidney), increasing background noise . Requires blocking steps (e.g., avidin/biotin blockers), complicating protocols .
- Fluorochrome-Tyramides (Cy3, Cy5, Fluorescein): Enable multiplexing with multiple fluorophores but exhibit lower sensitivity than enzymatic detection (e.g., Cy5-TSA showed minimal signal in BMP2 detection) . Limited by photobleaching and lower resolution in dense tissues .
TNP-Tyramide :
Multiplexing Capability
- DIG-tyramide is ideal for sequential labeling with fluorochrome-tyramides. For example, DIG-TSA with AP detection can be followed by Cy3-TSA for a second target without signal overlap .
- Biotin-tyramide is less suitable for multiplexing due to cross-reactivity with endogenous biotin .
Cost and Protocol Efficiency
DIG-Tyramide :
Fluorochrome-Tyramides :
TNP-Tyramide :
Comparative Data Table
Key Research Findings
DIG-TSA vs. Radioactive Probes :
- DIG-TSA with AP detection achieved comparable sensitivity to radioactive methods in BMP2 mRNA localization, eliminating radiation hazards .
Biotin vs. DIG in miRNA Detection :
- DIG-labeled LNA probes with TSA showed 10-fold higher sensitivity than biotin-labeled probes due to lower background .
Fluorochrome-TSA Limitations :
- Cy5-TSA underperformed in BMP2 detection, highlighting the trade-off between multiplexing and sensitivity .
Q & A
Basic Research Questions
Q. What methodological steps are critical for incorporating Digoxigenin Tyramide into fluorescent in situ hybridization (FISH) protocols?
- Answer : this compound is integral to signal amplification in FISH. Key steps include:
Probe Labeling : Riboprobes are labeled with digoxigenin (DIG) using RNA labeling kits.
Hybridization : Co-hybridize DIG-labeled probes with target RNA/DNA in tissue sections.
Signal Amplification : Incubate with anti-DIG horseradish peroxidase (HRP) conjugates, followed by tyramide substrate (e.g., biotin, Cy3, or fluorescein tyramide) to deposit localized fluorescent signals .
Detection : Use fluorescence microscopy to visualize amplified signals.
- Table 1 : Key Parameters for FISH Using this compound
Q. How does Tyramide Signal Amplification (TSA) enhance sensitivity in molecular assays using this compound?
- Answer : TSA leverages HRP-conjugated antibodies bound to DIG-labeled probes to catalyze localized deposition of tyramide substrates, amplifying signals 100–1000× compared to direct labeling. Critical factors include:
- Substrate Selection : Fluorescein, Cy3, or Cy5 tyramides for multiplexing.
- Kinetics : Short tyramide incubation (5–10 min) to prevent background noise.
- Blocking : Use serum or BSA to reduce non-specific HRP binding .
- Table 2 : Comparison of Signal Amplification Methods
Method | Sensitivity | Key Advantage | Limitation | Reference |
---|---|---|---|---|
TSA | 100–1000× | Compatible with multiplexing | Requires precise timing | |
ELF (Enzyme-Labeled Fluorescence) | 100× | Photostable signals | Limited dynamic range |
Advanced Research Questions
Q. What experimental parameters should be prioritized to minimize non-specific binding in multiplex assays using this compound?
- Answer : Non-specific binding arises from cross-reactivity or over-amplification. Mitigation strategies include:
- Probe Design : Ensure high AF2 pLDDT scores (>80) and low backbone-RMSD (<2 Å) for target specificity .
- Atomic Depth Criteria : Exclude probes where DIG-linker atoms are buried (Rosetta atomic_depth <30) to improve accessibility .
- Sequential Amplification : Separate tyramide reactions for each probe to prevent HRP cross-activity .
- Table 3 : Probe Design and Optimization Guidelines
Parameter | Optimal Range | Impact on Specificity | Reference |
---|---|---|---|
AF2 pLDDT | >80 | Ensures structural accuracy | |
Backbone-RMSD | <2 Å | Reduces off-target binding | |
Rosetta ddG | <−30 | Favors stable probe-target binding |
Q. How can researchers validate the specificity of this compound-based probes in complex tissue samples?
- Answer : Validation requires:
Negative Controls : Omit primary probes or use scrambled RNA probes.
Competition Assays : Pre-incubate with excess unlabeled probes to block binding.
Cross-Verification : Compare with orthogonal methods (e.g., qPCR or CRISPR knockouts) .
Data Reproducibility : Replicate experiments across ≥3 biological samples and document in Supporting Information per journal guidelines .
Q. What are the best practices for integrating this compound with other labels (e.g., fluorophores, biotin) in co-localization studies?
- Answer : For co-localization:
- Spectral Separation : Use tyramides with non-overlapping emission spectra (e.g., Cy3 + Cy5).
- Order of Amplification : Prioritize lower-abundance targets first to prevent signal saturation.
- Blocking Steps : Apply avidin/biotin blocking between sequential TSA reactions .
- Table 4 : Multiplexing Workflow for Co-Localization
Properties
Molecular Formula |
C39H56N2O8 |
---|---|
Molecular Weight |
680.9 g/mol |
IUPAC Name |
6-[[2-[[(3S,5R,8R,9S,10S,12R,13S,14S,17R)-12,14-dihydroxy-10,13-dimethyl-17-(5-oxo-2H-furan-3-yl)-1,2,3,4,5,6,7,8,9,11,12,15,16,17-tetradecahydrocyclopenta[a]phenanthren-3-yl]oxy]acetyl]amino]-N-[2-(4-hydroxyphenyl)ethyl]hexanamide |
InChI |
InChI=1S/C39H56N2O8/c1-37-16-13-29(48-24-35(45)40-18-5-3-4-6-34(44)41-19-15-25-7-10-28(42)11-8-25)21-27(37)9-12-31-32(37)22-33(43)38(2)30(14-17-39(31,38)47)26-20-36(46)49-23-26/h7-8,10-11,20,27,29-33,42-43,47H,3-6,9,12-19,21-24H2,1-2H3,(H,40,45)(H,41,44)/t27-,29+,30-,31-,32+,33-,37+,38+,39+/m1/s1 |
InChI Key |
HDWVXJDRHANLFV-AMVRZWEMSA-N |
Isomeric SMILES |
C[C@]12CC[C@@H](C[C@H]1CC[C@@H]3[C@@H]2C[C@H]([C@]4([C@@]3(CC[C@@H]4C5=CC(=O)OC5)O)C)O)OCC(=O)NCCCCCC(=O)NCCC6=CC=C(C=C6)O |
Canonical SMILES |
CC12CCC(CC1CCC3C2CC(C4(C3(CCC4C5=CC(=O)OC5)O)C)O)OCC(=O)NCCCCCC(=O)NCCC6=CC=C(C=C6)O |
Origin of Product |
United States |
Disclaimer and Information on In-Vitro Research Products
Please be aware that all articles and product information presented on BenchChem are intended solely for informational purposes. The products available for purchase on BenchChem are specifically designed for in-vitro studies, which are conducted outside of living organisms. In-vitro studies, derived from the Latin term "in glass," involve experiments performed in controlled laboratory settings using cells or tissues. It is important to note that these products are not categorized as medicines or drugs, and they have not received approval from the FDA for the prevention, treatment, or cure of any medical condition, ailment, or disease. We must emphasize that any form of bodily introduction of these products into humans or animals is strictly prohibited by law. It is essential to adhere to these guidelines to ensure compliance with legal and ethical standards in research and experimentation.