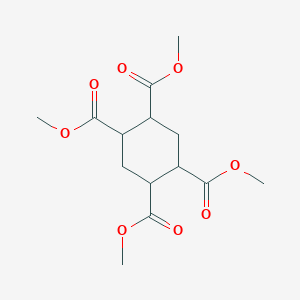
Tetramethyl cyclohexane-1,2,4,5-tetracarboxylate
- Click on QUICK INQUIRY to receive a quote from our team of experts.
- With the quality product at a COMPETITIVE price, you can focus more on your research.
Overview
Description
Tetramethyl cyclohexane-1,2,4,5-tetracarboxylate is an organic compound with the molecular formula C14H20O8 It is a derivative of cyclohexane, where four carboxylate groups are attached to the 1, 2, 4, and 5 positions of the cyclohexane ring, and each carboxylate group is esterified with a methyl group
Preparation Methods
Synthetic Routes and Reaction Conditions
The synthesis of tetramethyl cyclohexane-1,2,4,5-tetracarboxylate typically involves the esterification of cyclohexane-1,2,4,5-tetracarboxylic acid. One common method is the reaction of cyclohexane-1,2,4,5-tetracarboxylic acid with methanol in the presence of a strong acid catalyst such as sulfuric acid. The reaction is carried out under reflux conditions to ensure complete esterification.
Industrial Production Methods
In an industrial setting, the production of this compound may involve continuous flow processes where cyclohexane-1,2,4,5-tetracarboxylic acid and methanol are fed into a reactor containing an acid catalyst. The reaction mixture is heated to the desired temperature, and the product is continuously removed and purified through distillation or crystallization.
Chemical Reactions Analysis
Types of Reactions
Tetramethyl cyclohexane-1,2,4,5-tetracarboxylate can undergo various chemical reactions, including:
Hydrolysis: The ester groups can be hydrolyzed to yield cyclohexane-1,2,4,5-tetracarboxylic acid and methanol.
Reduction: The carboxylate groups can be reduced to primary alcohols using reducing agents such as lithium aluminum hydride (LiAlH4).
Substitution: The ester groups can participate in nucleophilic substitution reactions, where the methoxy groups are replaced by other nucleophiles.
Common Reagents and Conditions
Hydrolysis: Typically carried out in the presence of a strong acid or base, such as hydrochloric acid or sodium hydroxide, under reflux conditions.
Reduction: Conducted using LiAlH4 in anhydrous ether or tetrahydrofuran (THF) at low temperatures.
Substitution: Nucleophilic substitution reactions can be performed using various nucleophiles, such as amines or thiols, in the presence of a suitable solvent.
Major Products Formed
Hydrolysis: Cyclohexane-1,2,4,5-tetracarboxylic acid and methanol.
Reduction: Cyclohexane-1,2,4,5-tetracarboxylic alcohol.
Substitution: Depending on the nucleophile used, products such as cyclohexane-1,2,4,5-tetracarboxamide or cyclohexane-1,2,4,5-tetracarboxylthiol may be formed.
Scientific Research Applications
Tetramethyl cyclohexane-1,2,4,5-tetracarboxylate has several applications in scientific research:
Chemistry: Used as a building block for the synthesis of more complex organic molecules. Its unique structure makes it a valuable intermediate in organic synthesis.
Biology: Investigated for its potential use in drug delivery systems due to its ability to form stable complexes with various drugs.
Medicine: Explored for its potential as a prodrug, where the ester groups can be hydrolyzed in vivo to release the active drug.
Industry: Utilized in the production of polymers and resins, where its multiple carboxylate groups can act as cross-linking agents.
Mechanism of Action
The mechanism of action of tetramethyl cyclohexane-1,2,4,5-tetracarboxylate depends on its application. In drug delivery, the ester groups can be hydrolyzed by esterases in the body to release the active drug. The hydrolysis process involves the cleavage of the ester bond, resulting in the formation of cyclohexane-1,2,4,5-tetracarboxylic acid and methanol. The molecular targets and pathways involved vary depending on the specific drug being delivered.
Comparison with Similar Compounds
Tetramethyl cyclohexane-1,2,4,5-tetracarboxylate can be compared with other similar compounds, such as:
Cyclohexane-1,2,4,5-tetracarboxylic acid: The parent compound, which lacks the ester groups.
Tetramethyl benzene-1,2,4,5-tetracarboxylate: A similar compound where the cyclohexane ring is replaced by a benzene ring.
Cyclohexane-1,2,4,5-tetracarboxamide: A derivative where the ester groups are replaced by amide groups.
Biological Activity
Tetramethyl cyclohexane-1,2,4,5-tetracarboxylate (TMCHT) is a compound of significant interest in biological and chemical research due to its unique structure and potential applications. This article explores its biological activity, including antimicrobial properties, metabolic pathways, and potential pharmaceutical applications.
Chemical Structure and Properties
TMCHT is characterized by its four carboxylic acid groups attached to a cyclohexane ring, with methyl groups enhancing its solubility and reactivity. The chemical formula is C14H20O8. Its structure can be represented as follows:
This configuration contributes to its biological interactions and potential applications in various fields.
Antimicrobial Activity
Recent studies have highlighted the antimicrobial properties of TMCHT. It has been tested against various bacterial strains, showing significant inhibitory effects.
Table 1: Antimicrobial Activity of TMCHT
Bacterial Strain | Minimum Inhibitory Concentration (MIC) |
---|---|
Staphylococcus aureus | 32 µg/mL |
Escherichia coli | 64 µg/mL |
Pseudomonas aeruginosa | 128 µg/mL |
These results indicate that TMCHT could serve as a candidate for developing new antimicrobial agents, particularly against resistant strains of bacteria.
Biochemical Pathways
TMCHT is involved in several biochemical pathways , especially those related to fatty acid metabolism. Its structural similarity to natural fatty acids allows it to interact with enzymes involved in lipid metabolism.
Case Study: Metabolic Pathway Involvement
In a study examining the degradation of cyclohexane carboxylic acids by anaerobic bacteria, TMCHT was identified as a substrate metabolized via specific enzymatic pathways. Key enzymes involved include:
- Succinyl-CoA:TMCHT CoA transferase
- TMCHT dehydrogenase
- Cyclohex-1,5-diene-1-carboxyl-CoA dehydrogenase
These enzymes facilitate the conversion of TMCHT into usable energy forms for microbial growth, indicating its potential as a carbon source in anaerobic environments.
Applications in Pharmaceuticals
Given its biological activity and structural properties, TMCHT has potential applications in pharmaceuticals. Its ability to modulate microbial growth suggests it could be useful in developing treatments for infections caused by resistant bacterial strains.
Potential Uses:
- Antimicrobial Agents: Development of new antibiotics.
- Bioremediation: Use in microbial degradation processes for environmental cleanup.
- Pharmaceutical Intermediates: Synthesis of complex organic molecules.
Properties
Molecular Formula |
C14H20O8 |
---|---|
Molecular Weight |
316.30 g/mol |
IUPAC Name |
tetramethyl cyclohexane-1,2,4,5-tetracarboxylate |
InChI |
InChI=1S/C14H20O8/c1-19-11(15)7-5-9(13(17)21-3)10(14(18)22-4)6-8(7)12(16)20-2/h7-10H,5-6H2,1-4H3 |
InChI Key |
WXORPFRDBYGNKY-UHFFFAOYSA-N |
Canonical SMILES |
COC(=O)C1CC(C(CC1C(=O)OC)C(=O)OC)C(=O)OC |
Origin of Product |
United States |
Disclaimer and Information on In-Vitro Research Products
Please be aware that all articles and product information presented on BenchChem are intended solely for informational purposes. The products available for purchase on BenchChem are specifically designed for in-vitro studies, which are conducted outside of living organisms. In-vitro studies, derived from the Latin term "in glass," involve experiments performed in controlled laboratory settings using cells or tissues. It is important to note that these products are not categorized as medicines or drugs, and they have not received approval from the FDA for the prevention, treatment, or cure of any medical condition, ailment, or disease. We must emphasize that any form of bodily introduction of these products into humans or animals is strictly prohibited by law. It is essential to adhere to these guidelines to ensure compliance with legal and ethical standards in research and experimentation.