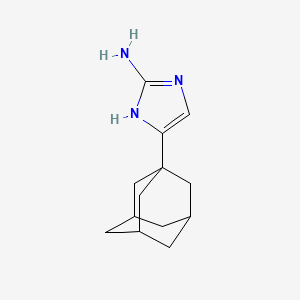
4-(adamantan-1-yl)-1H-imidazol-2-amine
Overview
Description
Synthesis Analysis
The synthesis of a similar compound, “(4-(Adamantan-1-yl)-1-(isopropyl)-1H-imidazol-2-yl)methanol”, was reported to be prepared through a five-step process starting from commercially available 1-acetyladamantane . Each step proceeded in moderate-to-excellent yields and the overall yield across five steps was 28% .Scientific Research Applications
Precursor for Biomimetic Chelating Ligands
The compound and its derivatives have the potential to be used as precursors to the synthesis of biomimetic chelating ligands . The imidazole groups are commonly incorporated into biomimetic model complexes designed to mimic the structure and/or function of metalloproteins .
Study of Reaction Pathways and Reactive Intermediates
The bulky adamantyl group in the compound could provide substantial steric shielding of a coordinated metal center. This steric shielding could promote the study of reaction pathways and reactive intermediates .
Anti-Dengue Virus Activity
The compound has shown anti-Dengue Virus (DENV) activity on all four serotypes in vitro via a mechanism that is speculated to involve inhibition of the uncoating step in the virus life cycle and/or blocking virus penetration into the host cell .
Synthesis of New Chelating Ligands
The compound, as well as its precursors, are potential starting materials for further elaboration into new chelating ligands .
Development of Anti-Viral Agents
The compound could be used in the development of agents that are able to inhibit the dengue virus (DENV), which is of utmost importance given the rapid spread of dengue fever in many tropical and sub-tropical regions .
Research in Bioinorganic Chemistry
The compound could be used in research in bioinorganic chemistry due to the propensity of the imidazole moiety to act as a donor ligand to metal atoms .
Mechanism of Action
Target of Action
A structurally similar compound, 1-(adamantan-1-yl)-2-(1h-imidazol-1-yl)ethanone, has been reported to target heme oxygenase 1 in humans . Heme oxygenase 1 is an essential enzyme that catalyzes the degradation of heme, a process that results in the formation of biliverdin, carbon monoxide, and free iron .
Mode of Action
Based on the known action of similar compounds, it can be hypothesized that 4-(adamantan-1-yl)-1h-imidazol-2-amine may interact with its target enzyme, potentially altering its function and leading to changes in downstream biochemical processes .
Biochemical Pathways
If the compound does indeed target heme oxygenase 1, it could potentially influence the heme degradation pathway . This could have downstream effects on various biological processes, including cellular respiration and the regulation of oxidative stress.
Pharmacokinetics
These properties are crucial for understanding the compound’s bioavailability, or the extent and rate at which the active moiety (drug or metabolite) enters systemic circulation, thereby accessing the site of action .
Result of Action
If the compound does indeed target heme oxygenase 1, it could potentially influence the levels of biliverdin, carbon monoxide, and free iron in the cell . These molecules have various roles in cellular function and could therefore lead to a range of downstream effects.
properties
IUPAC Name |
5-(1-adamantyl)-1H-imidazol-2-amine | |
---|---|---|
Source | PubChem | |
URL | https://pubchem.ncbi.nlm.nih.gov | |
Description | Data deposited in or computed by PubChem | |
InChI |
InChI=1S/C13H19N3/c14-12-15-7-11(16-12)13-4-8-1-9(5-13)3-10(2-8)6-13/h7-10H,1-6H2,(H3,14,15,16) | |
Source | PubChem | |
URL | https://pubchem.ncbi.nlm.nih.gov | |
Description | Data deposited in or computed by PubChem | |
InChI Key |
JKICBHBVAFWGNI-UHFFFAOYSA-N | |
Source | PubChem | |
URL | https://pubchem.ncbi.nlm.nih.gov | |
Description | Data deposited in or computed by PubChem | |
Canonical SMILES |
C1C2CC3CC1CC(C2)(C3)C4=CN=C(N4)N | |
Source | PubChem | |
URL | https://pubchem.ncbi.nlm.nih.gov | |
Description | Data deposited in or computed by PubChem | |
Molecular Formula |
C13H19N3 | |
Source | PubChem | |
URL | https://pubchem.ncbi.nlm.nih.gov | |
Description | Data deposited in or computed by PubChem | |
Molecular Weight |
217.31 g/mol | |
Source | PubChem | |
URL | https://pubchem.ncbi.nlm.nih.gov | |
Description | Data deposited in or computed by PubChem | |
Retrosynthesis Analysis
AI-Powered Synthesis Planning: Our tool employs the Template_relevance Pistachio, Template_relevance Bkms_metabolic, Template_relevance Pistachio_ringbreaker, Template_relevance Reaxys, Template_relevance Reaxys_biocatalysis model, leveraging a vast database of chemical reactions to predict feasible synthetic routes.
One-Step Synthesis Focus: Specifically designed for one-step synthesis, it provides concise and direct routes for your target compounds, streamlining the synthesis process.
Accurate Predictions: Utilizing the extensive PISTACHIO, BKMS_METABOLIC, PISTACHIO_RINGBREAKER, REAXYS, REAXYS_BIOCATALYSIS database, our tool offers high-accuracy predictions, reflecting the latest in chemical research and data.
Strategy Settings
Precursor scoring | Relevance Heuristic |
---|---|
Min. plausibility | 0.01 |
Model | Template_relevance |
Template Set | Pistachio/Bkms_metabolic/Pistachio_ringbreaker/Reaxys/Reaxys_biocatalysis |
Top-N result to add to graph | 6 |
Feasible Synthetic Routes
Disclaimer and Information on In-Vitro Research Products
Please be aware that all articles and product information presented on BenchChem are intended solely for informational purposes. The products available for purchase on BenchChem are specifically designed for in-vitro studies, which are conducted outside of living organisms. In-vitro studies, derived from the Latin term "in glass," involve experiments performed in controlled laboratory settings using cells or tissues. It is important to note that these products are not categorized as medicines or drugs, and they have not received approval from the FDA for the prevention, treatment, or cure of any medical condition, ailment, or disease. We must emphasize that any form of bodily introduction of these products into humans or animals is strictly prohibited by law. It is essential to adhere to these guidelines to ensure compliance with legal and ethical standards in research and experimentation.